Investigating neural oscillations in human brain organoids
Posted: 21 September 2021 | Anna Begley (Drug Target Review) | No comments yet
This article delves into research at the University of California Los Angeles, US, where stem-cell derived brain organoids that can mimic electrical activity have been developed.
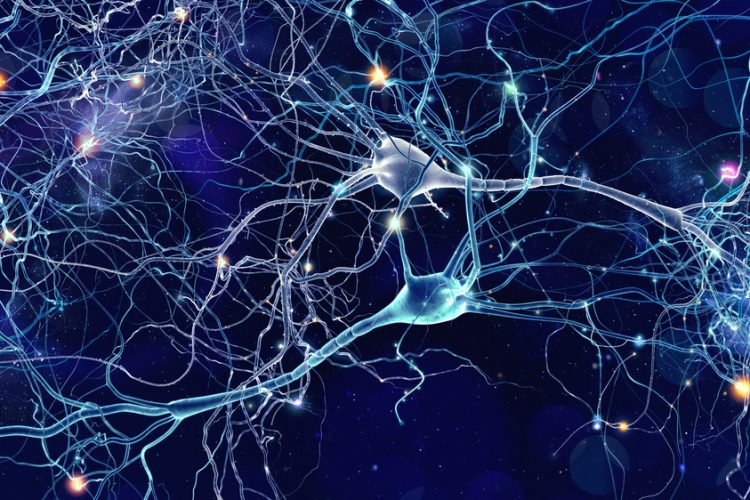
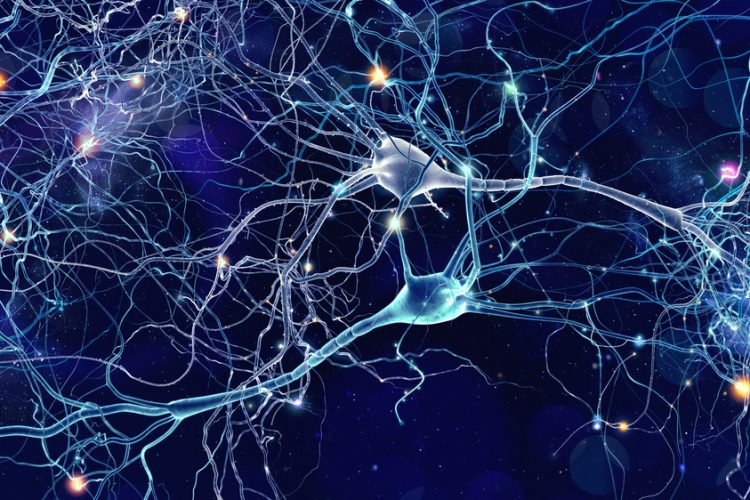
Over the past decade, researchers have discovered how to take cells from a person’s body, such as skin or blood cells, coax them in the lab to become induced pluripotent stem cells (iPSCs) and direct these to form any type of cell found in the body — including neurons. Scientists can encourage iPSCs to aggregate into three-dimensional forms, creating organoids that appear more like miniature human organs than cells in a flat dish. Including the use of kidney and lung organoids, this advance has enabled scientists to study a wide range of diseases.
The colossal challenges of brain organoid development
When it comes to the human brain, however, creating an organoid that mimics the organ’s structural complexity is especially difficult. “There are multiple challenges,” explained University of California Los Angeles (UCLA) researchers Professor Bennett Novitch and Assistant Professor Dr Ranmal Samarasinghe, in an exclusive with Drug Target Review. “First, organoid techniques are finicky and one must be very careful in conducting the experiments and ensuring that high quality control standards are applied. This is important for ensuring that the correct complement of cell types and structural organisation is reproducibly achieved to be able to accurately model neurological diseases.”
Both researchers added that brain organoids also develop along a timeline similar to the real human brain; it takes several months to create organoids that show robust neural network activities, making modelling the features of the mature/ageing brain particularly challenging. Furthermore, most protocols for making organoids generate a far more limited number of cells and regions than are found in human brains.
“Lastly, brain organoids are typically created without a connection to the blood system and without blood vessels and blood circulation, oxygenation and nutrient availability can be more limited in the organoids than it is in the real brain,” summarised Novitch and Samarasinghe. “This puts limits on both the size of the organoids that we can create, as the bigger the structures are, the harder it is to deliver nutrients into them and ensure that the organoid brain tissue remains healthy over time in culture.”
A scientific breakthrough
Facing these challenges, the UCLA team developed brain organoids derived from the skin cells of healthy individuals and investigated two different approaches to study the patterns of electrical activity inside them: one involved inserting a probe into each organoid to measure brain activity; the other entailed watching the brain cells in action under a microscope.
Some of the information they gathered was akin to the data scientists would find in electroencephalograms (EEGs). The analysis showed multiple kinds of neural oscillations. “I had not anticipated the range of oscillation patterns we would see,” commented Novitch. “By learning how to control which oscillation patterns an organoid exhibits, we may be able to eventually model different brain states.”
Mimicking Rett syndrome’s neural network
The team subsequently created brain organoids using cells from patients with Rett syndrome. “Rett syndrome is associated with a significant clinical phenotype including autism, learning disability and often epilepsy, but generally these patients do not have any obvious structural brain abnormalities by modern clinical imaging techniques, apart from some examples of microcephaly, but this is not consistent,” elaborated Novitch and Samarasinghe. “As such, Rett was an ideal disease to interrogate using our approaches. On a more practical note, we also had access to well-characterised, Rett patient iPSCs from two different patients, generated by our UCLA collaborators Drs William Lowry and Kathrin Plath.”


A microscope image of a mini brain organoid showing layered neural tissue and different types of neural cells. [credit: UCLA Broad Stem Cell Research Center/Nature Neuroscience]
The researchers began with patient-derived iPSCs and utilised protocols developed for generating brain organoids from these stem cells. They then created cortex-like organoids that have an abundance of excitatory cells and more ventral ganglionic eminence-like organoids that have interneurons or inhibitory cells.
“After we made these components, we physically combined them to make ‘fusions’. This approach creates a rich diversity of cell types, including both excitatory and inhibitory neurons and approximates the way that these neurons interconnect to form functional circuits in the body during embryonic development,” the researchers continued. “Having both excitatory and inhibitory neurons is critical for the emergence of higher-level network activities such as oscillatory rhythms, as we only saw these activities when we brought the cortex and ganglionic eminence organoids together.”
The team then used multiple approaches including extracellular recordings of local field potentials, whereby electrodes were placed directly into the organoids to measure electrical activity. They also used multiphoton-based calcium indicator imaging, involving the use of advanced microscopy to examine individual neural cell activity based on calcium release. Both techniques allowed the researchers to measure organoid neural circuit activity. In addition, they used immunohistochemistry and single cell RNA sequencing analysis methods to evaluate composition and structural organisation of the neural tissue within the organoids.
“Importantly, the strategy that we used to model neural network defects associated with Rett syndrome is very general and could be broadly applied to create analogous models for different neurological diseases starting with patient iPSCs or otherwise normal iPSCs engineered to carry disease-associated genetic mutations,” Novitch and Samarasinghe emphasised.
The medical potential of pifithrin-alpha
The researchers subsequently treated the Rett organoids with an experimental drug called Pifithrin-alpha. “Our studies show that Pifthrin-alpha suppresses (rather than elevates) seizure-like activities in our organoid model,” they explained. Pifithrin is a known repressor of the p53 tumour suppressor pathway which is associated with cellular stress responses and cancer. Prior research demonstrated that Pifithrin suppressed the expression of some of these cellular stress genes and normalised the structure of the neurons.
“We accordingly tested whether Pifithrin could alter the neural network abnormalities in our fusion organoid model for Rett syndrome,” said Novitch and Samarasinghe. “Remarkably, it suppressed some of the seizure-like activities that we had seen in Rett syndrome organoids and partially restored some of the lower frequency oscillatory rhythms. Pifithrin’s activities in these measures also appeared to be more efficacious than a traditional broad-spectrum antiseizure medication called valproic acid (VPA), which has been used to treat Rett patients.”
According to the researchers, the activation of cellular stress pathways is not unique to Rett syndrome and can be observed in neurons harbouring mutations in genes associated with other intellectual disabilities. This raises the possibility that Pifithrin or modulation of the molecular pathway(s) could be useful for treating a range of neurodevelopmental disorders.
“This is one of the first tangible examples of drug testing in action in a brain organoid,” concluded Samarasinghe. “We hope it serves as a stepping stone toward a better understanding of human brain biology and brain disease.”
The study was published in Nature Neuroscience.
Related topics
Drug Targets, Induced pluripotent (iPS) cells, Molecular Targets, Neurons, Neurosciences, Organoids, Protein, Small Molecules, Stem Cells, Target molecule, Targets
Related conditions
Rett syndrome
Related organisations
University of California Los Angeles (UCLA)
Related people
Dr Ranmal Samarasinghe, Professor Bennett Novitch