Better therapeutic antibodies by design
Posted: 21 June 2021 | Aparajita Dubey (CRAMbridge) | No comments yet
The binding specificity and high binding affinity of therapeutic antibodies makes them an ideal therapy for treating a wide range of human disorders. Advancements in antibody technologies have resulted in huge development success along with a boost in novel and improved treatment strategies. However, specific challenges and considerations are faced in designing, manufacturing and formulating antibodies, such as stability, bioavailability and immunological engagement. In this article, Aparajita Dubey evaluates the uses of therapeutic antibodies and the various forms they can take.
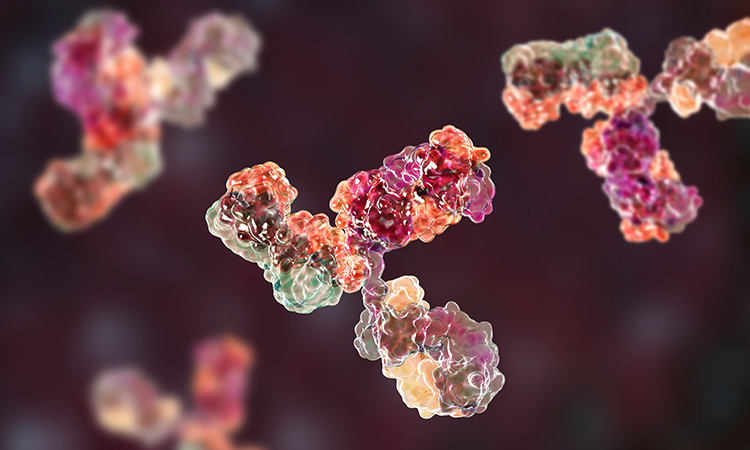
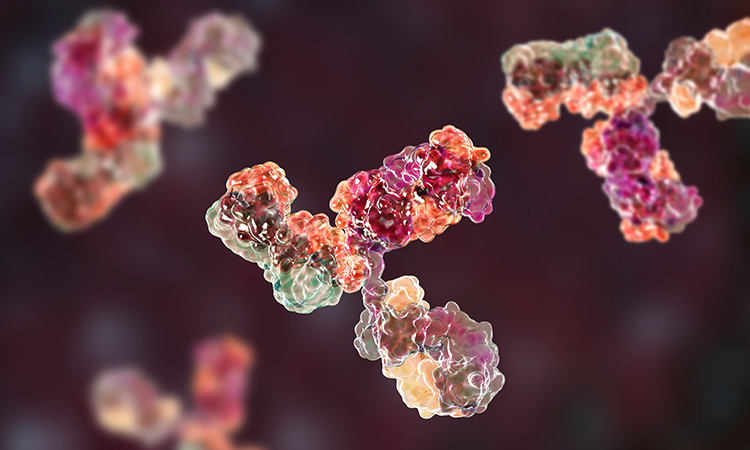
Since the approval of Orthoclone OKT3®, the first therapeutic monoclonal antibody (mAb) in 1986, this class of antibodies has been the most promising biologic therapeutic platform in the pharmaceutical industry. Antibodies have the potential to treat indications including cancers, infections, autoimmune disorders, cardiovascular and neurological diseases. These therapeutics are being applied and implemented to various newly identified biological targets and in different formats to produce strategically engineered next‑generation antibody therapeutics, termed “biobetters”.
These include different mAb formats such as antibody-drug conjugates (ADCs), bispecific antibodies (BsAbs), isotype-switched and glycoengineered antibodies. The objective of utilising these formats is to introduce exceptional potency, involve dual biological targets and modulate crystallisable fragment (Fc) effector functions. Different fragments of mAbs such as the Fc, antigen‑binding fragment (Fab) and single-chain variable fragment (scFv) possess defined functions and provide specificity to a biological target or immunological activation. Hence, these fragments are isolated and fused with other mAb fragments, biologically functional proteins, cytotoxic drugs or drug carriers and play a pivotal role in the next generation of biobetter therapies.
mAb developmental challenges and considerations
For therapeutic mAb development, a mammalian expression system is ideal for producing a biologically functional product. In contrast, for the development of a recombinant fusion mAb or a mAb fragment lacking glycosylation, lower organism expression systems can be considered. The glycosylation present in the conserved region of the Fc contributes to the stability and immune effector functions of the antibodies. A cell-based expression system is costly, sometimes inconsistent in yield and requires downstream processing to remove biological contaminants introduced during the expression. Affinity chromatography is a robust technology for mAb purification and other downstream processes such as viral inactivation. However, it involves extreme pH and salt conditions, which can chemically degrade the mAb quality, leading to product loss and instability.
There has been a lot of development in the field of bioprocess engineering and cell line development which have been extremely crucial in enhancing the manufacturing capabilities of therapeutic mAbs. Usually, the Chinese hamster ovary (CHO) cell line is used for commercial mAb expression, using a dihydrofolate reductase or glutamine synthetase stability selection system through resistance to methotrexate and methionine sulfoximine inhibition, respectively. Suspension culture supports higher cell densities for large‑scale fed‑batches, perfusion systems or continuous culture systems to achieve the required high titres for mAb production. Superior cell lines with enhanced metabolic functions, introducing glycosylation pathways, superior secretion and resistance to apoptosis can sustain a continuous bioprocess system. Various genetic engineering tools like vector design and highly efficient transcription, secretion, selection and integration elements, along with codon optimisation, are done to maximise mAb expression.
Protein A-ligand-based affinity chromatography is the most robust, efficient and frequently used mAb capture technology, with the advantage of integrating a viral inactivation step by holding the low pH-eluted mAb product before further purification steps. However, this exposes the mAb product to low pH at high concentrations, inducing mild instability.
Strategies to improve the formulation of mAbs are crucial with excipient and PEGylation technologies introduced to help circumvent transportation limitations specifically for pulmonary delivery. Several drug carrier technologies such as microencapsulation, liposome and nanoparticle formulations inherently enhance the stability and control of the release of mAbs, which can prolong their half-life. Subcutaneous formulation is preferable over intravenous formulation for next-generation therapies. Injectable mAb formulations can be further improved with the use of excipients to increase solubility, reduce viscosity and enhance the stability of mAbs. Amino acid composition, structure and potential glycosylation in their variable complementarity-determining regions (CDRs) dramatically affect the stability profile of mAbs. The higher affinity to cellular targets results in the increased uptake, internalisation and catabolism of mAbs, which reduces antibody-dependent cellular cytotoxicity (ADCC) and increases mAb clearance rate. To improve the half-life of mAb fragments, PEGylation, strategic Fc mutation and glycosylation engineering enhance neonatal Fc receptor (FcRn) recycling. Recombinant technologies enable their fusion to mAb Fc to introduce FcRn recycling as a protection mechanism to further improve the stability and half-life of biotherapeutics. The modulation of mAb effector functions through isotype switching, glycoengineering and strategic mutations is beneficial for developing more effective mAb treatment strategies.
Computational and technological advancement in designing mAbs
Computational tools are helpful to improve stability, solubility and to check aggregation of mAbs. Deep learning in biology is crucial for cellular imaging, finding genomic connections, advancing drug discovery, characterisation and designing therapeutic drugs, including biologics such as antibodies. Advancements of in silico analysis for mAb sequencing, structure, conformation and other biological interactions are crucial for optimising mAb-based therapeutics.
For therapeutic mAb development, a mammalian expression system is ideal for producing a biologically functional product”
During the current pandemic, rapid and untraceable viral mutations are extremely dangerous. Hence, these fast machine learning (ML) models are being used to predict the possible inhibitory synthetic antibodies for SARS-CoV-2. In this approach, data for thousands of virus‑antibody sequences and their clinical patient neutralisation response were collected and trained in a ML model to predict the antibody response. After screening thousands of hypothetical antibody sequences, nine stable antibodies have been found to inhibit SARS-CoV-2.
Several technologies – such as mass spectrometry, X-ray crystallography, circular dichroism (CD), infrared (IR) and Raman spectroscopy, cryogenic-electron microscopy and nuclear magnetic resonance (NMR) spectroscopy – are very important for the characterisation, aggregation prediction and designing of mAbs. The strategic glycan addition in the CDR region of mAbs shows an advantage in terms of its solubility and stability with an extended half-life.
ADCs
Exploiting the specific binding properties of mAbs can improve the therapeutic index of cell-killing agents for treating cancer. With more than 65 ADCs currently in clinical evaluation, ADCs have emerged as a landmark for treating both haematologic malignancies and solid tumours. The major challenge involved in developing an effective ADC is selecting the target antigen to which the treatment binds. Factors such as the number of target molecules expressed on the tumour surface, rate of internalisation, route of intracellular trafficking and target specificity for antibody selection with the required intrinsic biologic activity, all influence the ADC design and activity.
Monoclonal and multispecific antibodies
mAb-based immunotherapy has emerged as the main component of cancer therapy aside from traditional approaches such as surgery, radiation and chemotherapy. Antibodies possess huge clinical advantages with a diverse set of favourable mechanisms of action, directly targeting tumour cells and promoting long-lasting anti-tumour immune responses. With all these multifaceted properties, mAbs have emerged as a powerful therapeutic platform for developing new cancer treatment strategies and current combination therapies.
ADCC and immune checkpoint blockades
Despite notable clinical successes, the therapeutic resistance of mAb therapy remains a major challenge. The clinical efficacy of mAbs can be increased by focusing on studies relating to their mechanisms of action. Some major findings include the role of ADCC in mediating mAb responses. Hence, engineering strategies that augment ADCC activity could emerge as a promising future approach. Clinical benefits can be further maximised by combining tumour‑targeted mAbs with immune checkpoint blockades (ICBs). Mutations of antibody targets along with associated signalling pathways act as biomarkers of mAb efficacy and resistance. Hence, future mAb treatment strategies must include inhibitors of alternative signalling pathways to develop resistance.
BsAbs
BsAbs are a leading drug class for cancer therapy and are currently generated via:
- chemical conjugation with crosslinkers
- somatic fusion of two hybridoma lines (quadroma)
- genetic (protein/cell) engineering.
Several new techniques and platforms have been introduced for BsAb generation in recent years. The significant difference lies in the number of antigen-binding sites, geometry, half-life in the blood serum and effector functions. BsAb mechanisms of action are broadly classified into the following four formats:
- Bi-specific T-cell engagers (BiTEs)
- dual-affinity retargeting antibodies
- homodimeric “knob-in-hole” antibodies
- trifunctional BsAbs.
Advancements in protein and gene engineering technology has resulted in more than 100 different BsAb formats, which are much more complex and diverse than mAbs.
There are several significant advantages of BsAb formats over monospecific antibodies. For example, they provide higher binding specificity since they interact with two different surface antigens. BsAbs enhance cytotoxicity and direct specific effectors of the immune system to target tumour cells. The use of BsAbs compared to combination therapy involving two monospecific drugs is more commercially viable in terms of cost of development and clinical trials.
Conclusion
New development methods for BsAb generation have resulted in various promising antibody derivatives which differ from natural IgG in terms of pharmacokinetics, blood serum half‑life, ability to penetrate tumours, size, valence and presence of Fc. BsAbs can exhibit a synergistic effect by blocking several biological pathways, which is impossible with monospecific antibodies. A combination of different development methods will result in BsAbs directed against various diseases involving simultaneous binding of several specific antigens. Theoretically, BsAb-based therapy could be a better option for treating various cancers, but requires proof from further research.
About the author
Aparajita Dubey has more than 12 years of experience in pharmaceutical research, mainly in cancer biology and immunotherapy, at Biocon, Panacea and Jawaharlal Nehru University. As a biologist she has broad experience in the field of cell line development, cancer immunotherapy, molecular biology along with medical writing and consulting experience. She has an MSc in life sciences and a PG Diploma in IP from National Law School University, Bangalore, India.
References
- Sifniotis V, Cruz E, Eroglu B, Kayser V. Current Advancements in Addressing Key Challenges of Therapeutic Antibody Design, Manufacture, and Formulation. Antibodies (Basel). 2019 Jun 3;8(2):36. DOI: 10.3390/antib8020036. PMID: 31544842;
PMCID: PMC6640721. - A Review of Deep Learning Methods for Antibodies Jordan Graves, Jacob Byerly, Eduardo Priego, Naren Makkapati, S. Vince Parish, Brenda Medellin, and Monica Berrondo
- Zahavi D, Weiner L. Monoclonal Antibodies in Cancer Therapy. Antibodies (Basel). 2020 Jul 20;9(3):34. DOI: 10.3390/antib9030034. PMID: 32698317; PMCID: PMC7551545.
- Sedykh SE, Prinz VV, Buneva VN, Nevinsky GA. Bispecific antibodies: design, therapy, perspectives. Drug Des Devel Ther. 2018; 12:195-208. Published 2018 Jan 22. doi:10.2147/DDDT.S151282
- Almagro JC, Daniels-Wells TR, Perez-Tapia SM, Penichet ML. Progress and Challenges in the Design and Clinical Development of Antibodies for Cancer Therapy. Front Immunol. 2018 Jan 4; 8:1751. Doi: 10.3389/fimmu.2017.01751. PMID: 29379493;
PMCID: PMC5770808. - Huang S, van Duijnhoven SMJ, Sijts AJAM, van Elsas A. Bispecific antibodies targeting dual tumorassociated antigens in cancer therapy. J Cancer Res Clin Oncol. 2020 Dec;146(12):3111-3122. DOI: 10.1007/s00432-020-03404-6. Epub 2020 Sep 28.
PMID: 32989604; PMCID: PMC7679314. - Sawant MS, Streu CN, Wu L, Tessier PM. Toward Drug-Like Multispecific Antibodies by Design. Int J Mol Sci. 2020 Oct 12;21(20):7496. DOI: 10.3390/ijms21207496. PMID: 33053650; PMCID: PMC7589779.
- Hafeez U, Parakh S, Gan HK, Scott AM. Antibody-Drug Conjugates for Cancer Therapy.
Molecules. 2020 Oct 16;25(20):4764. DOI: 10.3390/molecules25204764. PMID: 33081383; PMCID: PMC7587605. - Lee CC, Perchiacca JM, Tessier PM. Toward aggregation-resistant antibodies by design. Trends Biotechnol. 2013 Nov;31(11):612-20. DOI: 10.1016/j.tibtech.2013.07.002. Epub 2013 Aug 7. PMID: 23932102.
- Lu, RM., Hwang, YC., Liu, IJ., et al. development of therapeutic antibodies to treat diseases. J Biomed Sci 27, 1 (2020). https://doi.org/10.1186/s12929-019-0592-z
- Sheridan, C. Bispecific antibodies poised to deliver a wave of cancer therapies. Nat Biotechnol 39, 251–254 (2021). https://doi.org/10.1038/s41587-021-00850-6
- Magar R, Yadav P, Barati Farimani A. Potential neutralizing antibodies discovered for novel coronavirus using machine learning. Sci Rep. 2021 Mar 4;11(1):5261. DOI: 10.1038/s41598-021-84637-4. PMID: 33664393; PMCID: PMC7970853.
- Finlay WJJ, Lugovskoy AA. De novo discovery of antibody drugs – great promise demands scrutiny. MAbs. 2019 Jul;11(5):809-811. DOI: 10.1080/19420862.2019.1622926. Epub 2019 Jun
Related topics
Antibodies, Biologics, Biopharmaceuticals, Monoclonal Antibody, Protein, Proteomics
Related organisations
Sartorius, Thermo Fisher Scientific