Revealing the genetic signature of neurodegenerative disease
Posted: 21 July 2020 | Hannah Balfour (Drug Target Review) | No comments yet
Which immune cells contribute towards immune-induced neurodegeneration and how could this knowledge enable conditions such Alzheimer’s and multiple sclerosis to be treated?
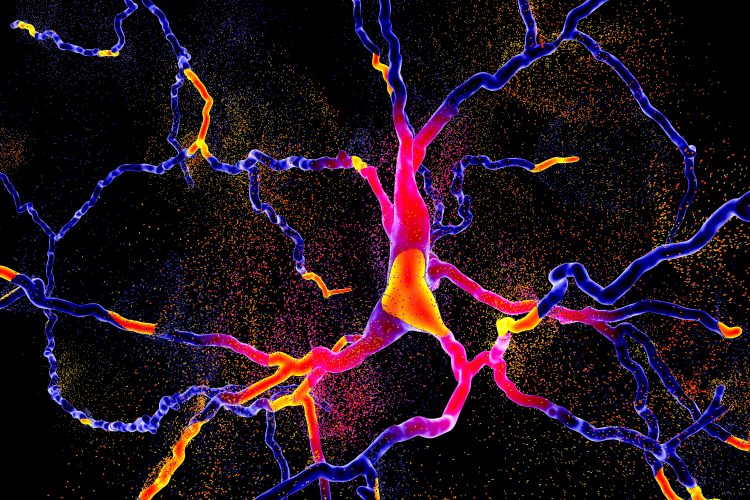
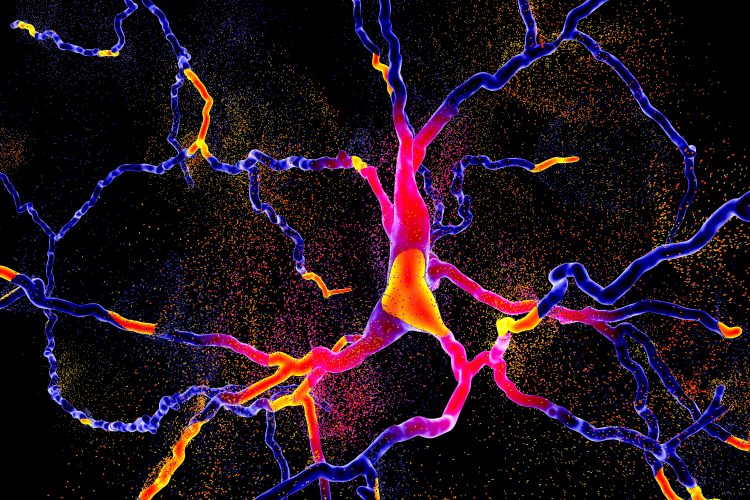
Neurodegenerative diseases are a growing burden on global health resources and the World Health Organization (WHO) estimates that by 2030, neurological disorders will account for approximately 12 percent of annual deaths.
With the scale of neurological conditions growing, especially in countries with increasing ageing populations, researchers have been attempting for decades to establish the causes of neurodegeneration and identify therapeutically beneficial drug targets in an attempt to develop therapies that can either prevent the onset of neurodegeneration or halt disease progression. The former therapeutic option is highly complicated, as disease onset for many neurodegenerative conditions occurs years prior to clinical symptoms appearing. As such, therapeutics to slow disease progression, and possibly reverse clinical symptoms, have been the focus of a huge volume of studies.
While it has been accepted for years that the immune system plays a role in degenerative diseases of the nervous system, it is still not understood which cells were implicated and their exact contribution to immune-induced neurodegeneration. Here, Drug Target Review’s Hannah Balfour discusses how a specific genetic signature expressed by immune cells signals their involvement in neurodegeneration and the possible druggable pathways this expression pattern reveals with Dr Katerina Akassoglou, Senior Investigator at the Gladstone Institutes and Professor of Neurology at the University of California, San Francisco.
Dr Akassoglou explained that in neurodegenerative diseases, such as Alzheimer’s disease (AD), Parkinson’s and multiple sclerosis (MS), the innate immune cells of the brain secrete reactive oxygen species (ROS), toxic substances that neurons are highly sensitive to. A healthy brain does not have high level of reactive oxygen species but, in the case of MS and AD, it is thought an oxidative burst – a sudden release of ROS taking over the environment – causes neurons and myelin-producing cells, called oligodendrocytes, to die off. This is called an oxidative injury.


Macrophage under the microscope.
Prior to Dr Akassoglou and colleagues sequencing the immune cells of the brain, it had never been established which immune cells contributed to oxidative injury and what their relative contribution was. To address this, the team developed Tox-seq and applied it in a murine model of MS. Tox-seq integrates single-cell RNA-sequencing technology with selective labelling of cells that produce oxidative stress to reveal which genes are being expressed. “While we knew many immune cells have the machinery to produce ROS, it was macrophage cells – peripheral immune cells that enter into the brain when there is disease – that were the major source identified,” she explained. In their research published in Nature Immunology, the Gladstone Institutes found over 80 percent of the cells expressing genes for the secretion of ROS were macrophages that had entered the brain.
Another contributor was one specific population of microglia, called MgV. Only this type of microglia had the machinery to produce ROS. Dr Akassoglou explained that when the genetic profile of these cells was analysed using Tox-seq and compared to that of the macrophages, a similar subset of genes was identified in both, which the researchers termed the ‘oxidative stress gene signature’.


A microglial cell stained with Rio Hortega’s silver carbonate method under the microscope.
Within this gene signature three primary categories of genes were co-expressed: oxidative stress genes, genes relating to the glutathione pathway (which regulates reduction and oxidation reactions) and genes that promote blood coagulation.
The protein fibrin, an essential factor for blood clotting, was identified by the team as an activator of microglial cells. This led them to screen potential compounds to target the genes implicated in this pathway. Of the 1,907 anti-inflammatory compounds screened, 128 supressed microglial activation. However, the team were unable to identify which impacted oxidative stress signalling. To address this, the researchers overlaid the oxidative stress gene signature with published drug target pathways and identified one drug, acivicin, which had activity against the γ-glutamyl transpeptidase (GGT) and oxidative stress genes in the signature.
Acivicin is a cancer drug that inhibits GGT, the enzyme that degrades glutathione – an antioxidant that neutralises ROS. Therefore, the team hypothesised acivicin may decrease oxidative stress by blocking glutathione degradation. When they treated in vitro cells that had the MS gene signature with acivicin, microglial activation and the release of ROS was blocked. Furthermore, when it was tested as a prophylactic for neurodegeneration in a murine model of MS, the same results were observed.
The researchers also experimented on mice with established disease; waiting for their neurodegeneration to progress 80 days before acivicin treatment, drugs are typically tested after only 12-20 days when the disease is mild. The researchers observed that the drug reversed the effects of progressive and chronic MS. Of note, there is currently no approved drug that has this effect in humans. Following treatment with acivicin, the mice had reduced paralysis, decreased inflammation and a profound decrease in the oxidative stress markers, to the point where Dr Akassoglou said the oxidative stress signature was “almost abolished”.
The researchers are hopeful that drugs could pharmacologically manipulate specific targets within the gene signature, or those populations of cells which express it, rather than supressing the whole immune system.
Dr Akassoglou said she hopes to continue leveraging Tox-seq and overlaying the resulting gene expression patterns with known drug-target pathways to identify if this gene signature is unique to MS or if it is more broadly expressed across different types of neurodegeneration, such as Alzheimer’s, Parkinson’s and traumatic brain injury (TBI). She said that a critical future discovery would be whether the gene signature is expressed across a range of conditions, as this would inform whether one therapeutic could be used to combat many neurodegenerative diseases. Alternatively, they may identify unique drug targets for each disease if the expression patterns are different.
Interestingly, Dr Akassoglou said that the GGT gene they identified as part of the gene signature has previously been tested as a biomarker in Alzheimer’s disease, suggesting there is some overlap between the expression of genes linked to oxidative stress in MS and Alzheimer’s.
a critical future discovery would be whether the gene signature is expressed across a range of conditions”
She concluded that one of the most surprising findings was that immune cells were expressing genes that promote coagulation. Fibrinogen is a coagulation factor that circulates in the blood and is a precursor to fibrin, the final product of the coagulation cascade. Upon injury, fibrinogen is converted to fibrin via activation of coagulation causing blood to clot. Fibrin is also deposited in tissues, a process that activates the immune system.
In their previous work, Dr Akassoglou and colleagues have shown that brains of patients with MS and Alzheimer’s have vascular abnormalities or damage with fibrin deposits that, when taken together with the later study, may suggest vascular injury initiates oxidative stress. Further damage to vasculature due to this state could be creating a vicious cycle, where oxidative stress, coagulation and vascular pathology each positively feed back onto one another, driving degeneration.
Dr Akassoglou said that understanding how vascular changes activate the immune system in neurodegenerative diseases would be a further important future discovery in this emerging field.
Dr Katerina Akassoglou is a Senior Investigator at the Gladstone Institutes and a Professor of Neurology at the University of California (UC) San Francisco. She is also the Director of the Gladstone Center for In Vivo Imaging Research. Katerina has pioneered studies on the mechanisms that control the communication between the brain, immune and vascular systems.
Related topics
Analytical techniques, Disease research, Drug Targets, Genetic analysis, Genomics, Immunology, In Vivo, Neurosciences, Technology
Related conditions
Alzheimer's disease (AD), Multiple Sclerosis (MS), Neurodegenerative diseases, Parkinson's disease (PD), Traumatic brain injury
Related organisations
Gladstone Institutes, University of California (UC) San Francisco
Related people
Dr Katerina Akassoglou