A novel approach to unravelling the mysteries of Alzheimer’s
Posted: 16 December 2019 | Dr Sean Devenish (Fluidic Analytics), Professor Tuomas Knowles (University of Cambridge) | No comments yet
The heterogenous and dynamic nature of protein aggregates makes them a particularly challenging class of structures to study. In this article, Professor Tuomas Knowles and Dr Sean Devenish present a novel approach to studying protein structures that could aid in understanding the complexities of Alzheimer’s disease and identify future therapeutic targets.
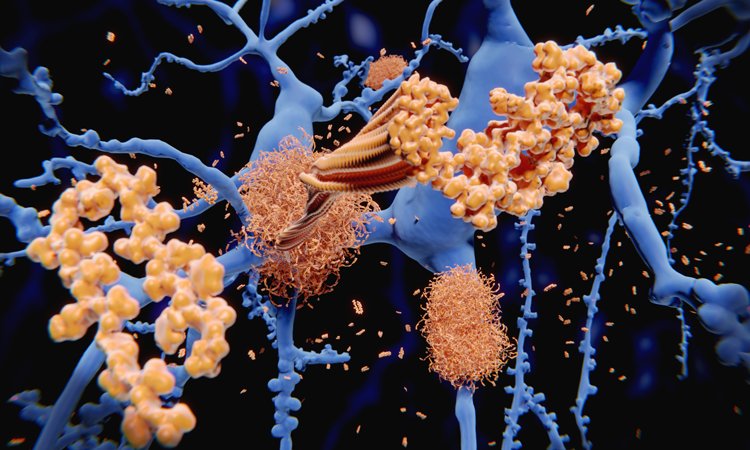
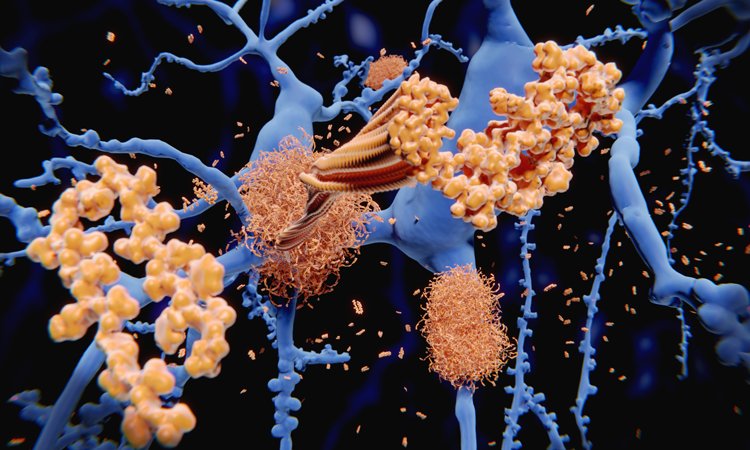
THE MOLECULAR machinery of living cells is largely built from protein molecules. These components interact to form complexes that drive most cellular functions. In many cases, proteins find their correct partners and self-assemble into their intended structures without difficulty. In rare cases, however, proteins may bind to an incorrect partner, or to the correct partner but in an incorrect manner, resulting in the formation of aberrant complexes, which are, in most cases, associated with disease. One of the most dramatic manifestations of this type of protein misassembly is the formation of amyloid aggregates in Alzheimer’s disease. With nearly 10 million new cases every year – one every three seconds – dementia is already a public health priority and with cases set to triple and reach 152 million by 2050,1 new experimental methods to understand the aberrant molecular structures formed in diseases such as Alzheimer’s are critical to develop new drugs.
With diffusional sizing, we were able to confidently generate accurate and complete in-solution data using amyloid proteins to connect stoichiometry and binding affinities with protein self-assembly”
This ‘molecular sociology’ of proteins2 – ie, who interacts with whom – is central to both biological function and, crucially, malfunction, but remains challenging to probe using conventional experimental techniques. Specifically, because protein aggregates are highly heterogeneous and dynamic, conventional methods for studying protein structure and function can be particularly challenging to apply to this class of structures. This is particularly true when investigating interactions of other species with protein aggregates – processes that are crucial for understanding molecular mechanisms of disease and when probing the interactions of candidate compounds with specific aggregated targets in drug discovery. Traditional methods like surface plasmon resonance (SPR) – often the de facto method for studying protein-protein interactions – require one of the binding partners to be attached or embedded to a surface. For proteins or peptides with a high level of disorder, such as amyloid beta (Aβ) or for aggregates like amyloid fibrils, this immobilisation is difficult to carry out and can yield unreliable results. This is because the immobilisation can affect the conformation of the peptide and the functionalisation of surfaces with a homogeneous preparation of aggregates is challenging to perform in a controlled manner.
Here, we look at a fundamentally new approach to studying interactions with complex targets, such as protein aggregates, and highlight recent results where these approaches have yielded novel insights into the role of natural protective mechanisms – molecular chaperones – present in cells that target protein aggregates. These chaperone systems keep protein aggregation at bay for most of our lifespans, therefore, an increased understanding of their modes of action can provide inspiration for developing new therapeutic compounds that mimic their action.
Genes, proteins and disease
Genes in DNA encode protein molecules that fold into precise three-dimensional, soluble structures capable of performing a specific task and function within a human cell. This central dogma is well known, but protein misfolding breaks these laws and challenges this understanding.
Misfolding of normally soluble peptides and proteins converts functional molecules into aggregates such as amyloid fibrils, a process that can also generate highly toxic oligomeric intermediates during the misassembly process. Amyloid fibrils and plaques found in the brains of patients with Alzheimer’s disease are mainly composed of a peptide called Aβ, which comes in two main isoforms: a 40- and 42-residue sequence. The peptides are derived from a protein called amyloid precursor protein (APP), which can be cleaved by beta secretase and gamma secretase to generate Aβ peptide. While the normal biological function of Aβ is not fully understood, its role in Alzheimer’s disease pathology has been intensely studied, but also remains challenging to fully understand.
Interestingly, there is increasing evidence that nature has evolved molecules capable of preventing this misbehaviour of the Aβ peptide, and indeed other peptides and proteins prone to form aggregates, namely molecular chaperones. Initially, the role of this species was thought to relate to aiding newly synthesised proteins to fold. It is increasingly apparent, however, that these molecules have key roles in recognising misfolded proteins, preventing their further proliferation and targeting these aberrant species for degradation. Molecular chaperones are thus central elements of the cell’s quality control mechanisms. There has been increasing interest in understanding how they can recognise specifically misfolded proteins and probing their modes of binding, with a view to learning key guiding principles of nature’s own defence systems against protein aggregation and taking inspiration from this in the search for therapeutics against these disorders.
Only by unravelling the complex network of protein interactions at play in health and disease, can targeted treatments be developed that stand a chance of succeeding”
However, probing interactions of other species with protein aggregates has proved to be particularly challenging. Indeed, these aggregates are challenging to prepare in a well-defined manner and attach to a surface; conditions that are required for the use of textbook methods for probing protein-protein interactions such as SPR. Moreover, the aggregates are typically too large to be able to move through the pores of matrices used in gel electrophoresis and their molecular weight is too high to be able to be ionised readily for mass spectrometry experiments. This situation has left the community of researchers conducting vitally important work in industry and academia on protein aggregates without a convenient tool to study their interactions in a quantitative manner.
Studying challenging proteins: the future?
The ability to study protein interactions in solution, in their native environments and without having to immobilise the molecules onto a surface increases the range of potential targets that can be investigated and opens up the possibility of performing quantitative binding studies and analysis on target classes, which were previously out of reach for such characterisation. Complex targets and aggregated proteins that can be challenging to attach to surfaces or embed in gels can be studied if the measurements can be performed under native conditions in solution, including disordered proteins that do not have defined globular structures and therefore are susceptible to perturbations when attached to surfaces. In addition, looking at proteins in their near-native environments can produce more realistic and true-to-life results, giving researchers confidence in their findings.
Microfluidic diffusional sizing (MDS) is a new method of protein analysis developed by a spinout from the University of Cambridge (Figure 1). The method can be used to assess on-target protein interactions in solution and in crude biological backgrounds – opening up possibilities for detailed analysis of proteins in their near-native states and natural environments. This has implications both for research – to understand more about the molecular mechanisms of disease – and during drug discovery when designing potential therapies.


Figure 1: Measuring protein-protein interactions through the direct effect that such interactions have on the molecular weights of the species present in solution and hence their diffusion coefficients. The instrument measures the rate of diffusion of proteins under steady state laminar flow in a microfluidic chip and converts them to an effective molecular weight or size.
The native state protein interaction method has recently been applied to study the way molecular chaperones recognise and bind to protein aggregates. A recent paper in Science Advances3 demonstrates the power of diffusional sizing for probing protein aggregates. The study looked at two key naturally occurring chaperone molecules, clusterin and brichos, that aim to protect against the deleterious effects of protein aggregates. This study used diffusional sizing to better understand how these molecular chaperones recognise and bind to Aβ protein aggregates. Using this technique, researchers were able to generate quantitative data for this highly heterogeneous system, including binding constants, which have been challenging to obtain using conventional methods. The ability to characterise the sizes of both bound and unbound chaperones helped identify the major targets that they bind to, and demonstrated that these chaperones recognise with high affinity the aggregated forms of the Aβ peptide but do not bind tightly to the monomeric form. Moreover, the study showed that the sites to which the chaperones bind on the fibrils are distinct, revealing the existence of multiple different structural environments on the fibril surface (Figure 2).


Figure 2: Direct measurement Figure 2 of the binding of the molecular chaperone to amyloid fibrils of the Aβ peptide. Hydrodynamic radius of the bound population is much larger than that of a monomeric protein, indicating that the target recognised by this chaperone is the aggregated form of the Aβ peptide. The ability to perform measurement in solution allows the binding affinity to be measured quantitatively. Data reproduced from Scheidt T. et al. Secondary nucleation and elongation occur at different sites on Alzheimer’s amyloid-β aggregates. Science Advances, 5 (2019).
During the FEBS/EMBO Women in Science Award plenary lecture at FEBS 2019 in Krakow, Poland, Professor Sara Linse presented work on amyloid proteins and showed examples of this technique to probe interactions of protein aggregates. She said: “With diffusional sizing, we were able to confidently generate accurate and complete in-solution data using amyloid proteins to connect stoichiometry and binding affinities with protein self-assembly.” This work clearly demonstrates the intricacies and subtleties surrounding the complex nature of protein aggregation and the challenges associated with quantitative biophysical measurements in this complex, but vitally important, space. New technologies that enable the elucidation of precise molecular details – such as the interplay between two molecular chaperones involved in aggregate formation and inhibition – help researchers to piece together the bigger picture. There has never been a more exciting time to work in protein science, and the potential offered by the introduction of new methods into this area is vast. More generally, technological advances have defined in the past progress in life sciences, as summarised by one of the pioneers of developmental biology, Sydney Brenner: “Progress [in science] depends on the interplay of techniques, discoveries and new ideas, probably in that order.” Only by unravelling the complex network of protein interactions at play in health and disease, can targeted treatments be developed that stand a chance of succeeding and the ability to shed new light on this problem using measurements that allow such processes to be probed under native conditions in solution opens up a new more true-to-life window into complex biomolecular systems.
About the authors
References
- https://www.who.int/mental_health/neurology/dementia/infographic_dementia.pdf?ua=1
- Robinson CV, Sali A, Baumeister W. The molecular sociology of the cell Nature, 450(7172):973-82 (2007)
- Scheidt, T. et al. Secondary nucleation and elongation occur at different sites on Alzheimer’s amyloid-β aggregates. Science Advances, 5 (2019)
Related topics
Disease research, Drug Targets, Neurons, Neurosciences, Pathology & Molecular Medicine, Research & Development, Targets
Related conditions
Alzheimer’s disease
Related people
Sydney Brenner