Mapping neurons to improve Parkinson’s disease therapies
Posted: 21 April 2016 | Victoria White, Digital Content Producer | No comments yet
Researchers have mapped out the pathways of a set of neurons responsible for the kinds of motor impairments found in patients with Parkinson’s disease…
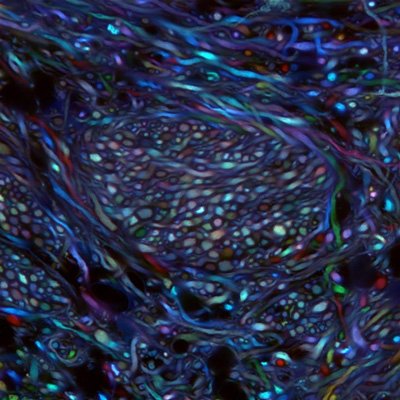
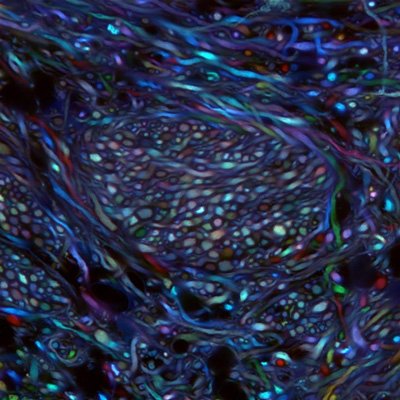
Using two laboratory techniques pioneered in part at Caltech, Caltech researchers have mapped out the pathways of a set of neurons responsible for the kinds of motor impairments found in patients with Parkinson’s disease.
In patients with Parkinson’s disease, gait disorders and difficulty with balance are often caused by the degeneration of a specific type of neuron – called cholinergic neurons – in a region of the brainstem called the pedunculopontine nucleus (PPN). Damage to this same population of neurons in the PPN is also linked to reward-based behaviours and disorders, such as addiction.
Previously, researchers had not been able to untangle the neural circuitry originating in the PPN to understand how both addictions and Parkinson’s motor impairments are modulated within the same population of cells. Furthermore, this uncertainty created a barrier to treating those motor symptoms. After all, deep brain stimulation can be used to correct walking and balance difficulties in these patients, but without knowing exactly which part of the PPN to target, the procedure can lead to mixed results.
“The circuits responsible for controlling our behaviours are not nicely lined up, where this side does locomotion and this side does reward,” says Viviana Gradinaru (BS ’05), assistant professor of biology and biological engineering.
Neurons are made up of a cell body and a long string-like axon that can diverge and project elsewhere into different areas of the brain. Because of this shape, the researchers realised they could follow the neuron’s “roots” to an area of the brain less crowded than the PPN. This would allow them to more easily look at the two very different behaviours and how they are implemented.
Researchers used optogenetics to manipulate neural activities
Cheng Xiao, a senior research scientist at Caltech, began by mapping the projections of the cholinergic neurons in the PPN of a rat using a technique developed by the Gradinaru lab called Passive CLARITY Technique, or PACT. In this technique, a solution of chemicals is applied to the brain; the chemicals dissolve the lipids in the tissue and render that region of the brain optically transparent and able to take up fluorescent markers that can label different types of neurons. The researchers could then follow the path of the PPN neurons of interest, marked by a fluorescent protein, by simply looking through the rest of the brain.
Using this method, Gradinaru and Xiao were able to trace the axons of the PPN neurons as they extended into two regions of the midbrain: the ventral substantia nigra, a landmark area for Parkinson’s disease that had been previously associated with locomotion; and the ventral tegmental area, a region of the brain that had been previously associated with reward.
Next, the researchers used an electrical recording technique to keep track of the signals sent by PPN neurons, confirming that these neurons do, in fact, communicate with their associated downstream structures in the midbrain. Then, the scientists went on to determine how this specific population of neurons affects behaviour. To do this, they used optogenetics, which allows researchers to manipulate neural activities – in this case, by either exciting or inhibiting the PPN neural projections in the midbrain – using different colours of light.
Using the optogenetic approach in rats, the researchers found that exciting the neuronal projections in the ventral substantia nigra would stimulate the animal to walk around its environment; by contrast, they could stop the animal’s movement by inhibiting these same projections. Furthermore, they found that they could stimulate reward-seeking behaviour by exciting the neuronal projections in the ventral tegmental area, but could cause aversive behavior by inhibiting these projections.
“Our results show that the cholinergic neurons from the PPN indeed have a role in controlling both behaviours,” Gradinaru says. “Although the neurons are very densely packed and intermingled, these pathways are, to some extent, dedicated to very specialised behaviours.” Determining which pathways are associated with which behaviours might also improve future treatments, she adds.
Related conditions
Parkinson's disease
Related organisations
California Institute of Technology (Caltech)