Determining the structure of nanobodies using nuclear magnetic resonance spectroscopy
Posted: 23 September 2021 | Professor Gennaro Esposito (NYU Abu Dhabi) | No comments yet
Scientists at the New York University (NYU) Abu Dhabi have used nuclear magnetic resonance (NMR) techniques to determine the structure of a specific nanobody, Nb23. Drug Target Review’s Victoria Rees spoke with lead researcher Professor Gennaro Esposito to find out how their findings could lead to a better understanding of how this small protein, derived from antibodies occurring only in camelids and sharks, can fight diseases ranging from rheumatoid arthritis to lupus, psoriasis, lymphoma and breast cancer.
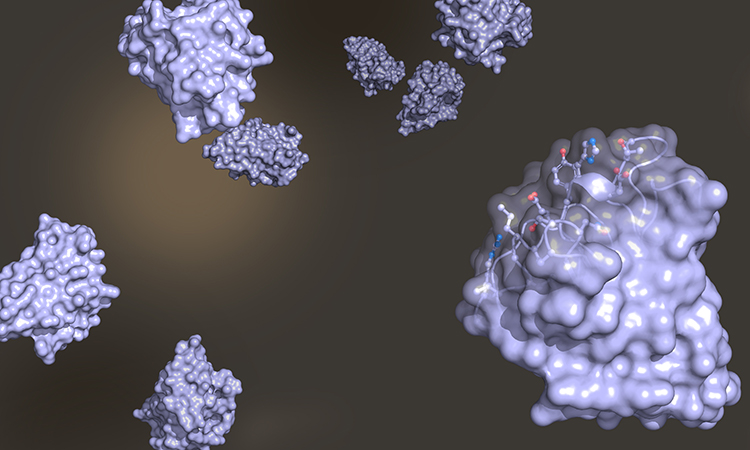
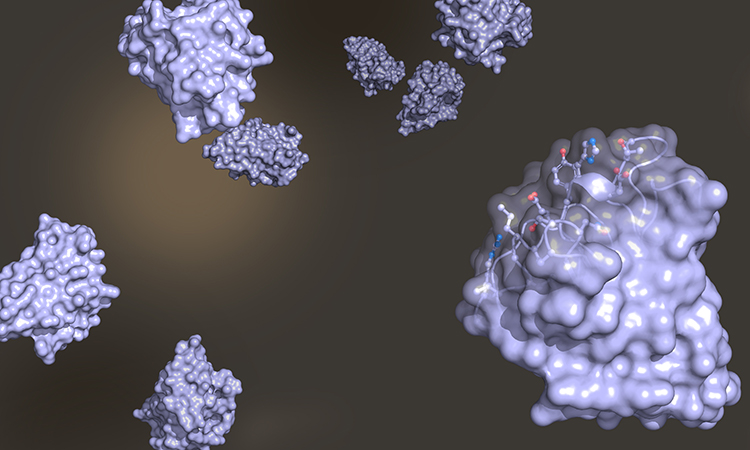
Why is it important to determine the structure of nanobodies?
Nanobodies are proteins derived from natural products, specifically from a class of antibodies found in camelids and sharks. Nanobodies are a promising class of therapeutics attracting significant interest because their performance can be “refined” via biotechnology and/or molecular engineering.
We need to know the structure of nanobodies to determine in detail what happens when a nanobody binds to its target as there are structural changes that may partially favour or disfavour the binding. Studying the structure of the nanobody, when it changes as a consequence of binding to the target, aids our understanding of the driving forces of this binding. In turn, this may contribute to the modification of nanobodies to improve their therapeutic performance.
Therefore, knowing the structure of nanobodies, before and after they bind, can suggest how to improve their modes of interaction.
Why did you focus on nanobody 23 (Nb23)?
We have been studying the formation of protein fibrils – the process underlying pathologies called amyloidoses. When proteins start to change their structure, because of chemical or surrounding ambient changes, they gather together and form bundles that eventually precipitate out, usually accumulating in extracellular space. This aggregate of proteins is commonly regarded as dangerous because it can impair intercellular communication by blocking the pores of the cells, altering the equilibria that determine homeostasis and the correct status of a cell. The consequences can be highly detrimental for the cells of the nervous system or any other tissue.
Nanobodies are a promising class of therapeutics attracting significant interest because their performance can be “refined” via biotechnology and/or molecular engineering”
Diseases like Alzheimer’s and Parkinson’s, for instance, are generated by this type of precipitation, the formation of amyloid, which is capable of affecting the intercellular communication of neurons. This can have devastating consequences on the capability of an individual to move, think, speak, etc.
Adding a nanobody that is capable of sequestering the proteins that are going to precipitate and form amyloid aggregates constitutes a tool to clear the environment from these potentially dangerous proteins. This is why we have been focusing on Nb23; it was originally prepared against a variant of beta2‑microglobulin, the protein responsible for a tissue-specific amyloidosis leading to amyloid deposits in joints of patients who undergo dialysis because of kidney dysfunction. However, there are naturally occurring mutants of this protein that are responsible for a much more diffuse (systemic) amyloidosis that affects organs like the intestine and liver. This type of disease is just one of those that nanobodies can address. Others include rheumatoid arthritis, lupus, psoriasis, lymphoma, breast cancer and more.
Recently, we have seen proposals of nanobodies against the Spike (S) protein of COVID-19 and our group, in collaboration with Professor Piergiorgio Percipalle, is currently studying a couple of nanobodies against a non-structural protein of COVID-19 that may be employed as a diagnostic tool and possibly as an antiviral to complement vaccines for the eradication of the virus responsible for the current pandemic.
Why did you use nuclear magnetic resonance (NMR) spectroscopy in your study?
NMR is a really important tool for the determination of protein structure in solution. It has been used for this type of study for almost 40 years. In fact, NMR is one of the only techniques, together with X-ray crystallography and nowadays cryo-electron microscopy, that can offer a picture of a molecule at the atomic level. Studying structures in a liquid state enables one to observe the proteins with the mobility and actual shape they adopt in the biological environments, whereas X-ray crystallography and cryo-electron microscopy look at crystals and frozen samples, respectively. In addition, NMR also allows us to see dynamic processes in real time.
In our study, we dissolved our proteins in solution – water with some additives – to produce similar conditions as a biological vehicle. However, one could obtain NMR spectra of proteins in a solid state too.
From an NMR measurement, we get a picture of the signal frequencies from the individual atoms of the molecule, which, in the beginning, is quite a complicated picture. It produces a huge amount of information that must be deciphered, which takes time. We assign a signal to each atom of the molecule; ie, the individual hydrogens, nitrogens and carbons of the protein have their own unique signal, so that we can associate each signal to a specific position of the protein sequence. Once you have this type of correlation, you are capable of determining what happens and where it happens on the molecule.
What did your results show?
We were able to reveal how the nanobodies can recognise the antigen target. The way nanobodies work is very interesting because they are just a single domain that recognises the target. Normally, antibodies need a huge frame, namely a pair of heavy chains and a pair of light chains, to adopt the proper three-dimensional shape enabling four recognition domains to keep the position for binding the target. However, camelids and sharks developed another type of antibody in which there are only two heavy chains, each contributing the same recognition domain capable of binding the target. The isolated recognition domain represents the nanobody that binds the target without the rest of the heavy chain frame.


Figure 1: Overlay of the Nb23 structure family (left) obtained from NMR. A detail of the NMR spectrum is shown on the right with yellow ovals highlighting the signal (right) and the position (left) of two atoms from Valine 127.
The way this happens is through the elongation of a particular region of the nanobody that penetrates and optimally fits in the cavities of the target protein by virtue of its flexibility. This determines the way nanobodies successfully bind to substrates.
In the case of Nb23, we have this elongation, called CDR3, that has many structural options, in principle. However, we have found that there is a strong polar interaction capable of restricting those structural possibilities, which should facilitate the binding and stabilise the complex. If an interaction can restrict the structural freedom, the unfavourable energy contribution from loss of freedom upon binding is decreased, which explains why the binding is efficient.
We are now working on the complex of Nb23 to confirm this. This is a very important point to collect clues for engineering Nb23, thereby improving the natural sequence.
What are the next steps for this research?
We have other nanobodies of the same family, so we can go through the same process again. If we keep studying the nanobodies of this family, we could eventually formulate an optimal sequence working satisfactorily with any beta2-microglobulin variant. Hopefully, we can come out with a proposal that is verifiable and could be developed into a drug candidate.
How do you see the future of nanobody therapeutics?
The greatest advantage of nanobodies is the versatility of their binding modes that makes these molecules amenable to molecular engineering and opens very wide avenues for biotechnology-based approaches”
Nanobodies are the evolution of antibody therapeutics. Although there are diseases that are treated with antibodies and they are the natural tool that our organism uses against any external offense, antibodies are huge and very complex molecules. An antibody works very well when it is produced within a protected cellular environment. However, an antibody-based drug can pose a lot of problems because production, conservation, handling and administration are quite critical. Antibodies have a molecular weight of 150 kDa, whereas nanobodies are one tenth of this – at most 15 kDa – which overcomes most of the above challenges. Antibodies are about 42nm long, whereas nanobodies are only 4nm long. With the tenfold reduction of the molecular weight, this makes nanobodies far more soluble into liquid preparations that behave as solutions rather than colloids.
Using antibodies against solid tumours does not work satisfactorily; some studies estimate that only 0.01 – 0.001 percent of the injected antibody actually reaches the tumour tissues. This is not true with nanobodies. The nanobody can reach the target tissue after being injected intravenously or into muscles, or even administered by aerosol.
However, the greatest advantage of nanobodies is the versatility of their binding modes that makes these molecules amenable to molecular engineering and opens very wide avenues for biotechnology-based approaches.
Professor Gennaro Esposito graduated in Chemistry at Naples University in 1979. In 1982 he became NMR researcher at Eniricerche (ENI). In 1990 he moved to Udine University as a Biophysics researcher and then as Associate Professor of Applied Physics. In 2018 he joined New York University (NYU) Abu Dhabi as visiting professor of Chemistry. He has also worked as a visiting scientist in the UK (Oxford and London) and Switzerland (Lausanne) and visiting professor in France (Grenoble) and Japan (Osaka).
Related topics
Antibodies, Imaging, Nuclear Magnetic Resonance (NMR), Protein, Proteomics, Spectroscopy, Structural biology
Related conditions
Alzheimer’s disease, Breast cancer, Covid-19, Lupus, lymphoma, Parkinson's disease, Psoriasis, rheumatoid arthritis
Related organisations
NanoTemper Technologies, Sartorius
Related people
Professor Piergiorgio Percipalle