Genetic screening to predict the risk of future diseases
Posted: 2 December 2020 | Dr Pushpanathan Muthuirulan (Harvard University) | No comments yet
The use of genetic testing has had a positive impact on patient care, bringing abundant opportunities for diagnosis or predictions of future diagnoses. Pushpanathan Muthuirulan explains how the application of genetic screening can help to customise healthcare for individuals based on their unique genetic makeup.
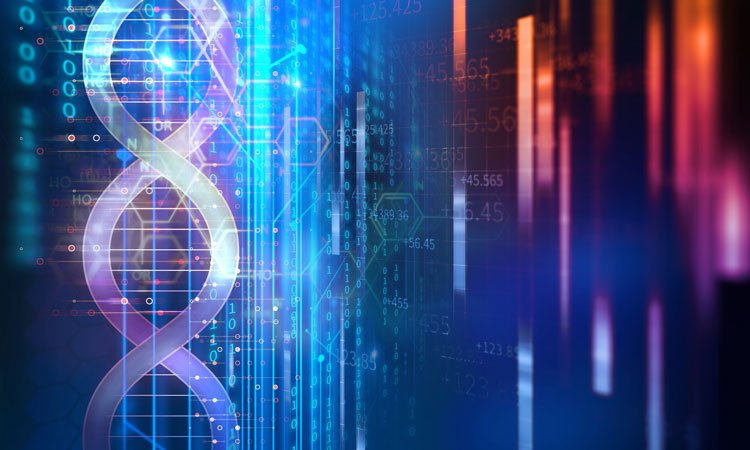
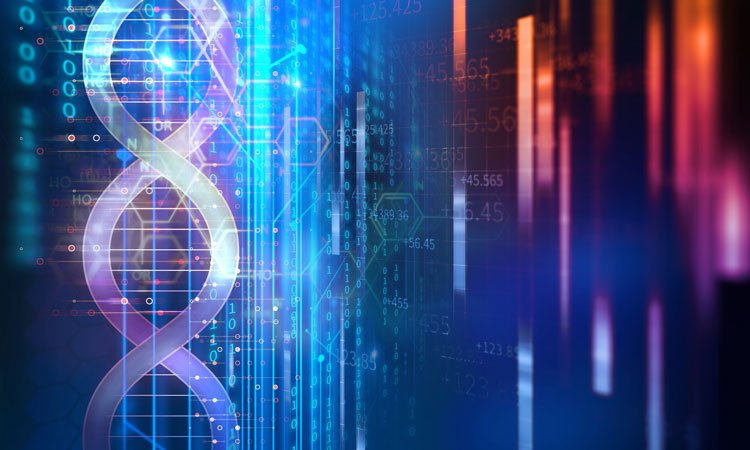
GENETIC SCREENING is an important tool for translating advances in genetics and genomics into public health benefits. It can test and characterise the genetic status of an individual who is suspected to be at increased risk for an inherited disease. Genetic screening methods have received a lot of attention in recent years and are becoming more mainstream and accessible to individuals and physicians. Given that no single screening method can detect all genetic conditions, each method should be individualised based on a person’s medical and family history.
Recent advances in molecular genetics and completion of the Human Genome Project (HGP) have brought phenomenal changes to clinical healthcare. They have paved the way for rapid improvements and the establishment of new technologies for early diagnosis and treatment of genetic diseases. Following the completion of the HGP, genetic screening approaches are progressively playing a key role in basic and translational research. Many early discoveries have contributed to important milestones in the discovery of new genetic factors and their impact on human life and diseases.
Genome Aggregation Database (gnomAD) has aggregated 15,708 whole genomes and 125,748 exome sequences. Analyses of these large datasets have created a catalogue of 241 million variants, which serves as a valuable resource for human genetics research and clinical variant interpretation.1 At a global scale, many millions of disease-relevant genes and genetic variants have been identified, which can be attributed to rare diseases and increased susceptibility to common chronic diseases, such as cancer and heart diseases. Currently, over 1,800 disease-relevant genes have been discovered and more than 2,000 genetic tests are available that can reliably test different genetic conditions in patients for early diagnosis and therapeutic intervention.
The risks for almost all human diseases arise from interactions between inherited genetic mutations and environmental factors. Individuals with more genetic predispositions are more likely to have increased risk of adverse health effects from environmental hazards than others.2
Genetic testing holds great promise and has made important strides in recent years”
Due to rapid advances in genomic technologies, genetic screening has become an essential tool in clinical practice and for disease research.6,7,8
In recent years, several new genetic screening methods have been developed for fast and reliable testing of genetic material, making it feasible to rapidly evaluate different genetic conditions that can guide clinicians in selecting the most appropriate disease prevention and treatment plans.
The different types of genetic screening include: newborn testing, diagnostic testing, predictive and pre-symptomatic testing, carrier testing, prenatal testing and preimplantation testing.
- Newborn screening is performed in infants after birth to identify genetic disorders that can be treated early in life. The infants are mostly tested for phenylketonuria (a genetic disorder that causes intellectual disability if left untreated) and congenital hypothyroidism (a disorder of the thyroid gland).9
- Diagnostic testing is performed to identify the genetic mutations or chromosomal abnormalities that cause diseases and, in most cases, is used to confirm diagnosis when a particular condition is suspected based on disease symptoms. Diagnostic testing is not available for all genes or genetic conditions and it can be performed just after birth or at any time during a person’s life.10
- Predictive and pre-symptomatic testing are used when no symptoms have yet developed, but there is a probability of carrying a mutation and developing disease later in life. Predictive testing can identify genetic mutations in a person developing disorders with a genetic basis, such as certain kinds of cancers, whereas pre-symptomatic testing can identify whether a person will develop a genetic disorder, such as hereditary haemochromatosis.11
- Carrier testing is relevant for people who carry a mutation and while not affected themselves, pose a risk to their offspring who may be affected. If both parents are tested, the test can provide information about a couple’s risk of having a child with a genetic condition.12
- Prenatal testing is important in families with known genetic disorders and it can detect changes in a foetus’s genes or chromosomes before birth that can help identify all possible inherited disorders and birth defects.
- Preimplantation testing is used to detect genetic changes in embryos and can reduce the risk of having a child with a particular genetic or chromosomal disorder.13 There is no single genetic screening method that can detect all genetic conditions. The different types of genetic screening methods available include HotSpot and comprehensive profiling, single-gene testing, deletion/duplication analysis, repeat expansion analysis, panel testing and large-scale genetic testing using next-generation sequencing (NGS).
- HotSpot profiling focuses on specific locations within a gene known to be associated with disease. It analyses specific gene areas without looking at the rest of the gene and often misses potentially actionable alterations.
- Comprehensive profiling looks at an entire gene to identify all possible alterations that may be driving disease development. It also expands on HotSpot testing to analyse both coding and non-coding sequences of the DNA to give a more comprehensive view of the genes.14
- Single-gene testing is performed to identify alterations or mutations in a single gene. This can be performed when people have symptoms of a specific condition or syndrome. Some examples of when single-gene testing is useful include for Duchene muscular dystrophy or sickle cell disease. Single-gene testing can also be used when there is a known genetic mutation in a family.
- Deletion/duplication analysis is used to evaluate partial or full gene deletions or duplications defined at the exon level. There are several categories of disorders that can be tested using deletion/duplication analysis, which include autosomal dominant developmental disorders caused by haploinsufficiency, multiple congenital anomaly syndromes, inherited (familial) cancer and genome instability syndromes, autosomal recessive metabolic disorders, X-linked intellectual disability, autism spectrum disorders, inherited cardiac disorders and inherited eye disorders.
- Repeat-expansion analysis is a preferred tool over standard sequencing methods for detecting small-scale repetitive nucleotide gains or losses in the genome, such as those seen in repeat disorders including Friedreich Ataxia (FRDA), Huntington Disease (HD), Gilbert-Meulengracht Disease, Myotonic Dystrophy Type 2 (DM2), etc.
- The panel genetic test is used to evaluate changes in many genes in a single test. Genetic testing panels are grouped in different categories based on different medical concerns. A few examples of genetic panel tests include low muscle tone, short stature and epilepsy. Panel genetic tests can also be grouped into those genes linked to a higher risk of developing certain kinds of cancer such as breast and colorectal.
- Large-scale genetic testing includes whole exome sequencing (WES) and whole genome sequencing (WGS). WES looks at all genes in the genome related to medical conditions (clinical exome), whereas WGS looks at all the patient’s DNA (including genes and non-coding sequences) simultaneously.
Due to rapid advances in genomic technologies, genetic screening has become an essential tool in clinical practice and for disease research”
All these genetic screening methods should be individualised based on a person’s medical and family history and what condition they are being tested for.15
Genetic testing holds great promise and has made important strides in recent years. However, these methods are still in their early stages and require further studies, not only to identify associations between genetic factors and treatment outcomes, but also to confirm preliminary findings, clarify the meaning of genetic associations and translate them into prescribing guidelines for disease prevention and treatment plans. With the application of genetic testing for personalised medicine, we can customise healthcare tailored to each individual based on their unique genetic makeup.
About the author
Dr Pushpanathan Muthuirulan is currently a Research Associate at Harvard University studying the developmental and genetic basis of human height variations using functional genomics approaches. Previously, he worked as a Postdoctoral Researcher at the National Institutes of Health, where his research focused on developing state-of- the-art technologies using CRISPR-Cas9 and super-resolution microscopy to map neural circuits that involves visual motion information processing in Drosophila. His expertise lies in omics technologies, drug discovery, neuroscience and developmental and evolutionary genetics.
References
- Karczewski K, Francioli L. (2017). The genome aggregation database (gnomAD). MacArthur Labs.
- Durmaz AA, Karaca E, Demkow U, Toruner G, Schoumans J, Cogulu O. (2015). Evolution of genetic techniques: past, present, and beyond. BioMed research international, 2015.
- Andermann A, Blancquaert I. (2010). Genetic screening: A primer for primary care. Canadian Family Physician, 56(4), 333-339.
- Clarke A. (1995). Population screening for genetic susceptibility to disease. BMJ, 311(6996), 35-38.
- World Health Organization. (2002). The world health report 2002: reducing risks, promoting healthy life. World Health Organization.
- Muthuirulan P. (2018). NGS: hunting mysterious ‘Dark Matter Genome’ towards rewriting the rules of human genetic diseases. Drug Target Review, 4(3), 72-75.
- Muthuirulan P. (2019). Genomic medicine: cracking genomes to cure ‘incurable’ diseases. Drug Target Review, Issue 3, 32-34.
- Muthuirulan P. (2020). Genomic assays: on the brink of revolutionising human healthcare. Drug Target Review, 7(1), 11-13.
- Rose SR, Brown RS. American Academy of Pediatrics & American Thyroid Association. (2006). Update of newborn screening and therapy for congenital hypothyroidism. Pediatrics, 117(6), 2290-2303.
- Creighton S, Almqvist EW, MacGregor D, Fernandez B, Hogg H, Beis J, MacKenzie J. (2003). Predictive, pre‐natal and diagnostic genetic testing for Huntington’s disease: the experience in Canada from 1987 to 2000. Clinical genetics, 63(6), 462-475.
- Borry P, Stultiëns L, Nys H, Cassiman JJ, Dierickx K. (2006). Presymptomatic and predictive genetic testing in minors: a systematic review of guidelines and position papers. Clinical genetics, 70(5), 374-381.
- Sherman S, Pletcher BA, Driscoll DA. (2005). Fragile X syndrome: diagnostic and carrier testing. Genetics in Medicine, 7(8), 584-587.
- District of Columbia Department of Health. (2010). Understanding genetics: a district of Columbia guide for patients and health professionals.
- Alvarez RH, Thomas JW, Chalmers ZR, Schrock AB, Tapias CA, Frampton GM, Markman M. (2016). Comparison of comprehensive genomic profiling (CGP) and hotspot next generation sequencing (NGS) assays in identifying treatment options for care of patients with metastatic cancer in in the community setting.
- Xue Y, Ankala A, Wilcox WR, Hegde MR. (2015). Solving the molecular diagnostic testing conundrum for Mendelian disorders in the era of next-generation sequencing: single-gene, gene panel, or exome/genome sequencing. Genetics in Medicine, 17(6), 444-451.
Related topics
Disease research, DNA, Gene testing, Genetic analysis, Genomics, Research & Development, Screening
Related organisations
Bio-Rad, Genome Aggregation Database (gnomAD) Consortium, Horizon Discovery, Human Genome Project (HGP)