Seeing the light – visualising protein translation in real time
Posted: 4 June 2020 | Nikki Withers (Drug Target Review) | No comments yet
Non-invasive imaging has played an increasing role in drug discovery and development processes. Nikki Withers spoke with Yochi Slonim, who discusses a novel technology that leverages FRET signals for the discovery of small molecule drugs that selectively control mRNA translation in a novel target space.
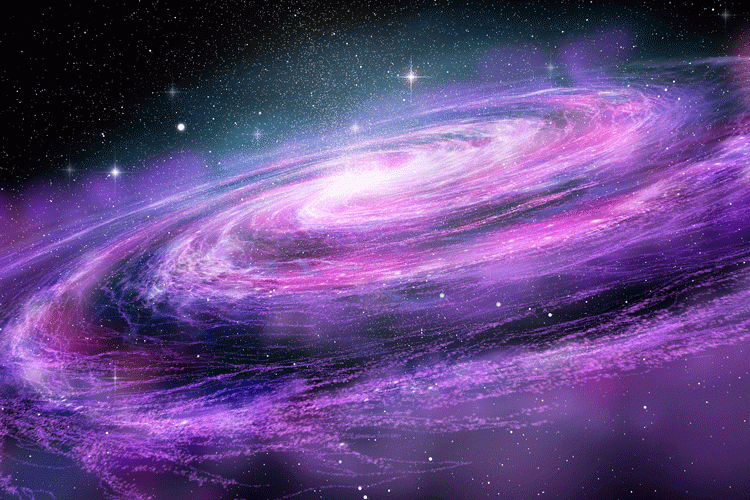
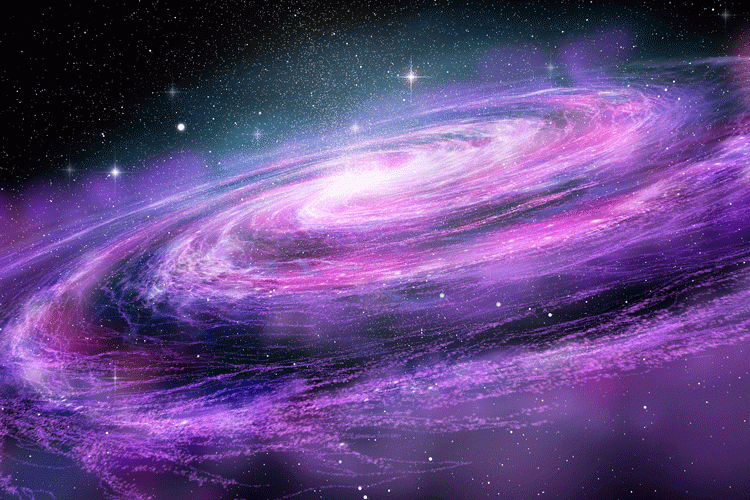
Drug development methods have traditionally relied upon molecules binding pockets on proteins’ surfaces – altering the function to treat a disease. However, many disease-causing proteins do not have such pockets and are therefore undruggable by conventional methods.
Fluorescence tools offer attractive options for visualising the effects of drug candidates in cells. These fluorescent tools, such as fluorescence resonance energy transfer (FRET), are regularly used in a range of drug discovery processes.
To address the shortcomings of traditional drug discovery methods, a novel approach for discovering drug candidates has been developed, which acts before a protein is made – during the process of protein synthesis. At the heart of the platform is new technology enabling visualisation of FRET light pulses from within ribosomes. “We are able to monitor mRNA translation of specific proteins or a group of proteins inside cells, visualising when, where and how much of a target protein is being made in a disease model in real time,” explained Yochi Slonim, co-founder and CEO of Anima Biotech.
A novel target space
Regulation of protein expression by targeting mRNA is already a validated approach in drug development, as indicated by RNAi-based therapeutics. However, these drugs are not small molecules which severely limits their use as a therapeutic strategy. Targeting mRNA secondary structures with small molecules is an emerging field, but the therapeutic outcomes of binding to mRNA with small molecules is not yet validated. Furthermore, this approach cannot achieve tissue selectivity since such drugs would bind to the target mRNA everywhere it is expressed.
By selecting the signature tRNA pair and transfecting it into cells the light that we will see will be highly correlated to the translation of that protein”
Slonim explained that his team has instead developed a high-content screening platform using fluorescently labelled transfer RNA (tRNA). tRNAs play a prominent role by transporting specific amino acids as per codes on mRNA to the ribosomes that chain amino acids into proteins. “Our idea was to attach fluorescent colours to tRNAs and transfect them into cells. When a pair of labelled tRNAs ends up sitting a ribosome, it generates a FRET signal, enabling us to see the light of protein translation in real time,” he said.
To achieve this, the team has purified and labelled the full repertoire of human tRNAs (46 isoacceptors) with fluorescent tags (Figure 1). Two complete tRNA libraries have been designed: one tagged with an energy donor and one tagged with an energy acceptor (red and green). “This is an amazing opportunity for generating FRET signals. The ribosome puts two tRNAs together, unloading the amino acids and building the protein chain. This is a repeatable event that happens in every ribosome, in every cell – it may be the most repeatable event that exists in nature. We can visualise this in images that look like the milky way at night,” he said.


Figure 1: Proprietary fluorescent labelled tRNA library.
Providing further examples of how this can be visualised, Slonim outlined the concept of transfecting all 46 labelled tRNAs into cells. “From time to time, you will have a pair of red and green labelled tRNAs sitting in a ribosome as part of the normal protein translation happening in cells and we will see the light,” he said. “This will happen for any combination of two tRNAs, for any labelled pair, so the light we see will be highly correlated with the total translation of mRNA.”
How is this useful in drug discovery?
To monitor the translation of a specific protein, Slonim described how the ‘signature pair’ of that protein needs to be selected. “Eighty-five percent of human proteins have a signature pair, a pair that is frequently used by ribosomes during their assembly but is almost unused when other proteins are being made. This is a fascinating bioinformatics fact that has no apparent reason,” he explained. “By selecting the signature tRNA pair and transfecting it into cells the light that we will see will be highly correlated to the translation of that protein. It means that we can individually monitor almost any protein’s translation.”
Figure 2 provides a real-time example of this approach. “If you look at the collagen I protein, there are frequent repeats of PG (proline, glycine) tRNAs in the sequence for assembly of the protein. This is codon specific so let us call them P1G1. We took that signature pair and transfected it into healthy lung fibroblasts, allowing visualisation of the light from within the ribosomes. This light is highly correlated with the translation of collagen I because the PG pair repeats frequently when the collagen I is being made but is almost unused by other proteins. The signal-to-noise ratio is 0.98, meaning that nearly all the light that you are seeing in this image comes from collagen I translation.” However, Slonim points out that in the healthy lung fibroblasts there is little collagen I being made: “Compare this to the next image of activated lung fibroblasts where you can visualise lung fibrosis happening with this enormous cloud of light. Collagen I is produced all over the place and you can visualise the disease happening in real time.”


Figure 2: Example: Collagen translation inhibitors for lung fibrosis.
From this, Slonim explains that his team can then screen for compounds that modulate the light. Figure 2 shows three compounds that came out of the screen that diminished the light to a great extent. These compounds are translation modulators and when they were used to treat these cells, they reduced the translation of collagen I and over five days the collagen I accumulation disappeared. “What we have here is a translation screen which is very visual,” explained Slonim, adding that they then perform a counter screen to ensure, for example, that the compounds are not general translation inhibitors. “To achieve this, we test these hits with all the 46 types of the tRNA. If the light goes off it means they are general translation inhibitors and should be ruled out.”
This novel target space enables the discovery of drugs that can selectively either decrease or increase the production of almost any given protein”
The researchers then perform hit validation and selectivity analysis: “We analyse the impact of hit compounds on overall protein synthesis, eliminating compounds that are toxic, that interfere with the synthesis of many of the cell’s proteins or that shut down the translation machinery itself. Additional translation selectivity assays have also been developed to monitor the effect of compounds on groups of proteins of interest, or specific proteins along a pathway. An important part of the platform is the elucidation of the mechanism of action of these compounds. Across several programmes in multiple therapeutic areas we have succeeded in deconvoluting the mechanism and ensured that they are selective translation inhibitors for the protein of interest.”
Opening a broad novel target space
Slonim concluded that the platform enables the identification of small molecules that selectively modulate the mRNA translation of target proteins. “This novel target space enables the discovery of drugs that can selectively either decrease or increase the production of almost any given protein. Moreover, because the mechanisms that regulate translation in cells tend to be tissue specific, we can discover drugs that work in a tissue selective manner, controlling the translation of the protein only in a specific tissue. This limits the off-target impact of drug candidates’ systemic effects on areas not affected by disease, essentially producing small molecule drugs that are much safer.”
The team are already working with Eli Lilly in a multi-year collaboration focusing on several undisclosed Lilly targets in the area of neuroscience. They are using their technology platform to discover lead candidates that are translation inhibitors of the targets. “We are excited to be collaborating with Lilly in the discovery of new drugs for some of the world’s most challenging diseases,” said Slonim.
Related topics
Analysis, Analytical techniques, Drug Discovery, Drug Targets, Imaging, Protein, Protein Expression, Proteomics, Research & Development, RNAs, Small Molecules, Technology
Related organisations
Anima Biotech, Molecular Devices
Related people
Yochi Slonim (Anima Biotech)