Realising the promise of laboratory automation in biomedical research
Posted: 11 April 2019 | Pushpanathan Muthuirulan - Harvard University Cambridge | No comments yet
Automation offers a choice of powerful ways to design and execute high-quality laboratory research. The use of lab automation is now pervasive in biomedical labs, offering versatile platforms on which to perform an ever‑expanding array of tasks free of human errors, and a unique means to address the problems associated with reproducibility – thus ‘bringing science to life’.
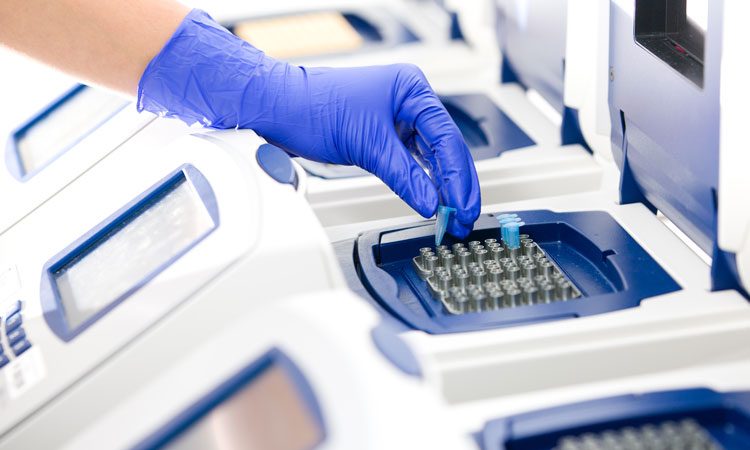
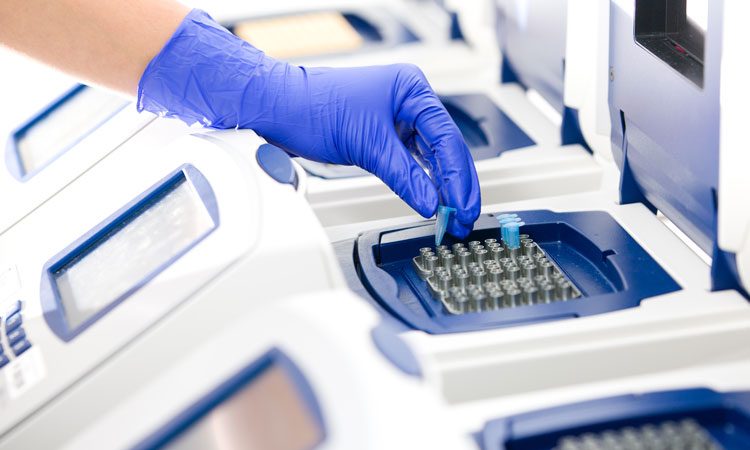
Laboratory automation is a multi-disciplinary strategy that integrates robotics, computers, liquid handling, and numerous other technologies. Lab automated systems can take many forms and perform numerous functions, but they are entirely built for those pursuing high-throughput screenings. Automation also enables the development of robust platforms and reliable workflows to generate reproducible data from new and complex scientific research methods with the goal of developing effective treatments and cures. The use of lab automation has become pervasive in biomedical research as it facilitates the invention of an array of devices that enable time savings and improved performance by eliminating human errors.1
Today’s scientists face a reproducibility crisis with their research; approximately 70 percent of researchers have tried and failed to reproduce another researcher’s experiment, and more than half have failed to reproduce their own. Lack of reproducibility in biomedical research can cause a significant delay in bringing life-saving and life-changing drugs, treatments and therapies to those who need it most.2 In addition, a recent study has shown that medical error is another major issue putting patients at risk and contributing to substantial unavoidable healthcare costs.3,4 In this context, lab automation can help biomedical scientists to achieve safety goals and address many of these challenges by improving the reproducibility and reliability of their experimental data, thus increasing the efficiency of their research. Lab automation not only enhances the productivity of research, but also increases reproducibility, accuracy and reduces human errors. We are also seeing this technology moving into the academic and industrial world, as biotech and pharma companies are outsourcing drug discovery pipelines and translational research workflows to academic drug discovery labs. Thus, continued advances in lab automation will enable scientists to develop high-value biomedical products, processes, technologies and services that would tackle the world’s health problems and help millions around the world to live better lives.
Lab automation is speeding up biomedical research
A major bottleneck in biomedical research is the lack of cross-disciplinary collaboration. Historically it has been difficult for researchers to perform tedious manual tasks whilst trying to remember settings on particular instruments. At the inception of lab technologies in the early 1980s, PCR amplification of DNA was a laborious and truly time-consuming process. Thermal cycling steps in PCR were carried out manually by researchers and involved repeated transfers of DNA samples among three large water baths set at different temperatures for denaturation, annealing and extension. In addition, scientists did not have heat-stable DNA polymerases and the enzyme had to be manually replenished after every PCR reaction.5 However, the ongoing escalation in resource cost and time led automation engineers to invent an all-in-one instrument called ‘thermal cycler’ to help automate this process.6 Since its introduction, significant improvements have been made to the instrument’s technical features, which have improved PCR experiments. The automation of PCR has paved the way for molecular biology research, and become an essential tool for scientists. In recent years, researchers have been deploying innovative technologies in their scientific research, including the use of robots to help them automate their workflows. In addition, current lab automation systems are made exceptionally easy to use by researchers with no prior experience in programming and robotics. Lab automation systems are also equipped with intuitive user interfaces that enable faster and more efficient protocol setups, and convenient access to instruments anytime and anywhere using a mobile device or desktop computer. Moreover, connectivity of equipment to the cloud-based system also offers freedom to create a shared protocol, start/stop, or to monitor the running status of the equipment.7 Essentially, the more advanced state of automation and robotics is revolutionising biomedical research and speeding up life science research by freeing scientists from performing tedious routine lab work.
Clinical microbiology laboratory automation
Laboratory automation systems are rapidly evolving and have been implemented in several diagnostic disciplines, such as microbiology, chemistry, haematology and molecular biology. In recent years, lab automation systems have proven their ability to enhance productivity and enable researchers to manage multiple clinical tasks despite a limited budget and personnel shortages. Medical laboratories often automate their workflow to gain productivity and reduce diagnostic errors. The automated systems – such as blood culture systems, automated microbial identification, and automated antimicrobial susceptibility testing systems – are widely utilised in microbiology laboratories to simplify collection and identification of specimens.8 However, the introduction of two technological innovations such as liquid-based transport devices and matrix-assisted laser desorption/ionisation time-of-flight mass spectrometry (MALDI-TOF) have changed attitudes about automation in microbiology. Liquid-based transport devices enable inoculation of the clinical specimen and smear preparation with automated liquid-based specimen processors,9 whereas MALDI-TOF systems allow accurate, rapid and inexpensive identification of microorganisms isolated from clinical specimens. MALDI-TOF processes are highly amenable to automation because they are technically simple and reproducible; particularly because the results do not change based on the organisms tested.10 There is, therefore, no doubt that implementation of laboratory automation in clinical microbiology laboratories has potential value – including the possibility of substantial cost savings, standardisation of initial sample processing, rapid microbial identification and antimicrobial susceptibility testing for earlier microbiological analysis and pathogen-directed treatment.
Automation of the molecular diagnostic laboratory
Molecular diagnostics have revolutionised the field of molecular medicine. Molecular diagnostics instruments have achieved total laboratory automation over time and enabled clinicians to more readily provide diagnostic results to healthcare providers, enabling them to deliver comprehensive evidence-based recommendations for the assessment and pharmacological treatment of patients with complex diseases. Implementations of lab automation in molecular diagnostic laboratories have considerably changed our routine laboratory life, particularly our medical practice and healthcare vision.11 In recent years, molecular diagnostic assays relied heavily on real-time PCR technology, which detects the amplification of DNA in real time while PCR is in progress by the use of a fluorescent reporter. Real-time PCR platforms are based on a simple automated workflow that circumvents most of the procedures used after a regular PCR reaction, such as gel electrophoresis or reverse-hybridisation. More than 75 percent of molecular diagnostic tests performed in clinics are amenable to real-time PCR procedures, and this technology has provoked a new molecular biology market by offering rapid assays for better diagnosis and personalised treatment of complex diseases.12 Additionally, the concept of integrating PCR technologies with other nucleic acid extraction platforms, such as fast magnetic beads-based procedures, has propelled molecular diagnostics to a new dimension of importance in the healthcare industry.11 Thus, there is no doubt that automation of the molecular diagnostics laboratory could greatly increase the rate of scientific discovery and help improve our understanding of biology, in the hope of developing new diagnostics, novel drugs and personalised therapies.
Lab automation of genomics workflows
Genomics has created new opportunities for drug discovery and enabled biomedical scientists to predict the risk of developing complex diseases, understand disease progression, and discover new drugs that specifically target the molecular pathway of diseases. A key driver for the genomics revolution has been the development of new high-throughput technologies and the automation of workflows for sample handling, genome interrogation, and data analysis. In recent years, automated laboratory systems have been built for a number of genomics applications, including DNA/RNA extraction, RNAi screening, CRISPR analysis and gene expression studies. Fully integrated automated systems offer a completely walk-away automation workflow for nucleic acid extraction, DNA normalisation, PCR plate preparation and real-time PCR assays. High-throughput, high-content RNAi screening systems offer tremendous opportunities for biomedical scientists to screen a compound library in a single run, and it becomes even more powerful when combined with a high-content imaging system that has provided valuable insight towards understanding gene function.13 RNA-based high-throughput expression analysis systems were designed to automate real-time PCR to facilitate high-throughput gene expression studies.14 Gene expression analysis and high-content screening (HCS) systems were designed to accommodate both gene expression analysis and perform HCS for genomics research.15
Besides these, automating sequencing library preparations (liquid handling, fragmentation, size selection) minimises errors, reduces handling time and enables high throughput.16 Therefore, all these platforms include key components to support genomics research and address the researcher’s flexibility requirements.
Conclusion
Lab automation represents an invaluable tool for biomedical research and development. Today’s scientists require an integrated and flexible automated platform to reduce human errors, enhance productivity and reproducibility of research. Implementation of lab automation technology in different biomedical sectors would enable scientists to generate better data and make confident decisions. Thus, advances in lab automation technology, coupled with a flexible screening platform, will lead to better diagnostic products and personalised management of complex diseases.
Biography
Pushpanathan Muthuirulan is currently a Research Associate at Harvard University studying the developmental and genetic basis of human height variations using functional genomics approaches. Previously, he worked as a Postdoctoral Researcher at the National Institutes of Health, where his research focused on developing state-of-the-art technologies using CRISPR-Cas9 and super‑resolution microscopy to map neural circuits that involves visual motion information processing in Drosophila. His expertise lies in omics technologies, drug discovery, neuroscience and developmental and evolutionary genetics.
Address of correspondence Harvard University, Cambridge, MA 02138 Phone: +1301-674-3108, Email: muthuirulanp@ fas.harvard.edu
References
1. Muthuirulan P.. Lab automation and robotics – accelerating the pace of antimicrobial therapy. Drug Target Review. 2018. 1(1), 6-8.
2. Baker M. 1,500 scientists lift the lid on reproducibility. Nature News. 2016. May 26;533(7604):452.
3. Makary MA, Daniel M. Medical error – the third leading cause of death in the US. Bmj. 2016. 353, i2139.
4. Van Den Bos J, Rustagi K, Gray T, Halford M, Ziemkiewicz E, Shreve J. The $17.1 billion problem: the annual cost of measurable medical errors. Health Affairs. 2011. 30(4), 596-603.
5. Rabinow P. Making PCR: A story of biotechnology. University of Chicago Press; 2011 Nov 27.
6. Weier HU, Gray JW. A programmable system to perform the polymerase chain reaction. Dna. 1988. 7(6), 441-447.
7. Olsen K. The first 110 years of laboratory automation: technologies, applications, and the creative scientist. Journal of laboratory automation. 2012. 17(6), 469-480.
8. Bourbeau PP, Ledeboer NA. Automation in clinical microbiology. Journal of clinical microbiology. 2013. JCM-00301.
9. Gizzie N, Adukwu E. Evaluation of Liquid based Swab Transport Systems against the New Approved CLSI M40-A2 Standard. Journal of clinical microbiology. 2016. JCM-03337.
10. Singhal N, Kumar M, Kanaujia PK, Virdi JS. MALDI-TOF mass spectrometry: an emerging technology for microbial identification and diagnosis. Frontiers in microbiology. 2015. 6, 791.
11. Merel P. Perspectives on Molecular Diagnostics Automation. JALA: Journal of the Association for Laboratory Automation. 2005. 10(5), 342-350.
12. Bustin SA. Developments in real-time PCR research and molecular diagnostics. Expert review of molecular diagnostics. 2010. 10(6), 713-715.
13. Krausz E. High-content siRNA screening. Molecular bioSystems. 2007. 3(4), 232-240.
14. Pinhasov A, Mei J, Amaratunga D, Amato FA, Lu H, Kauffman J, Ilyin SE. Gene expression analysis for high throughput screening applications. Combinatorial chemistry & high throughput screening. 2004. 7(2), 133-140.
15. Buchser W, Collins M, Garyantes T, Guha R, Haney S, Lemmon V, Trask OJ. Assay development guidelines for image-based high content screening, high content analysis and high content imaging. 2014.
16. May M. Automated sample preparation. Science. 2016. 351(6270), 300-302.
Related topics
Analysis, CRISPR, DNA, Genomics, High Throughput Screening (HTS), Microbiology, Polymerase Chain Reactions (PCRs), Screening