Pharmacological targeting of mitochondrial dysfunction in Parkinson’s disease: approaches and perspectives
Posted: 19 September 2018 | Iryna Karaban, Iryna Mankovska, Kateryna Rozova, Larysa Bratus, Olga Gonchar | No comments yet
Mitochondrial dysfunction has been widely implicated in the neuronal degeneration in Parkinson’s disease (PD). Mitochondria-targeted protective compounds that prevent or minimise a wide range of mitochondrial defects constitute potential therapeutic strategies in the prevention and treatment of neuronal degeneration in PD.
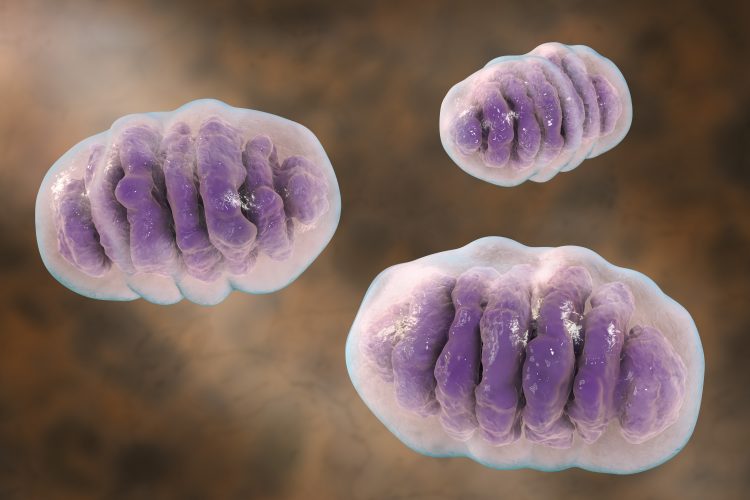
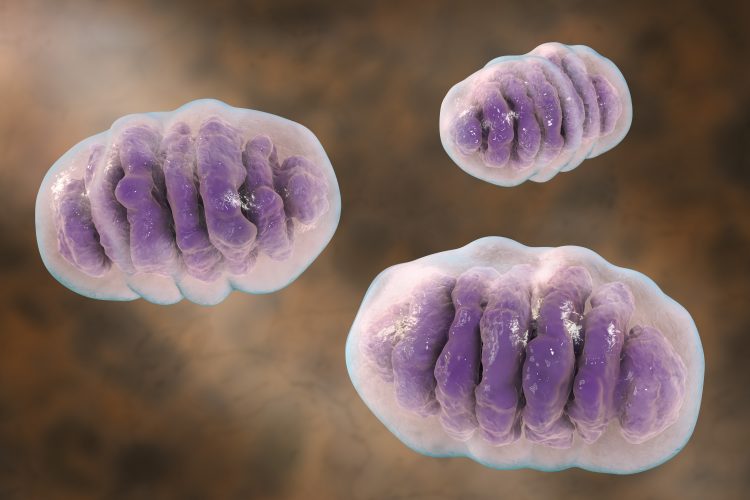
This review discusses the latest findings in this field obtained in PD patients and in animal and cellular models of PD with focus on the effects of pharmacological agents on mitochondrial biogenesis, fission, fusion, mitophagy machinery, and transcription of endogenous cytoprotective antioxidant enzymes. We also present data concerning the technologies for research and screening of novel bioactive molecules to target mitochondrial dysfunction in Parkinson’s disease.
PD is a progressive age-related neurodegenerative disease characterised clinically by presenting ‘TRAP’ signs (tremor, rigidity, akinesia and postural instability).1 The pathological hallmarks of PD include preferential loss of dopaminergic (DA) neurons in the substantia nigra pars compacta (SNpc) of the brain and α-synuclein containing inclusions in neurons throughout the brain.2 It is now firmly established that dysfunctional mitochondria and oxidative stress are crucial in Parkinson’s disease (PD) pathogenesis.1 Pharmacological agents targeting mitochondrial dysfunction and oxidative stress are prime candidates for neuroprotection in PD. The clinical prospect of effective gene therapies for the primary mitochondrial disorders connected with a mutation in mitochondrial DNA (mtDNA) or in nuclear genes encoding mitochondrial proteins in PD remains distant. The secondary mitochondrial dysfunction caused by the events that originate outside mitochondria is a promising therapeutic target.2 Parkinson’s disease is an example of a neurodegenerative disease having common patterns of cell disruption due to both primary and secondary mitochondrial dysfunction.1 It was noted that mitochondrial dysfunction is emerging as a common feature, suggesting a great pathogenic overlap among the familial, environmental and even sporadic forms of PD, with a potential convergence at the level of mitochondrial quality control.3,4 Pharmacological intervention can be used to treat PD patients with primary and secondary mitochondrial disorders.5 There are at least three general pharmacological strategies for ameliorating mitochondrial dysfunction:2
- Targeting bioactive molecules to mitochondria
- Modulating druggable mitochondrial targets and processes
- Affecting mitochondria indirectly by pharmacological agents acting on the transcription of mitochondrial genes.
The first strategy focuses primarily on the area of bioactive molecules to the mitochondria.6 Lipophilic cations such as triphenylphosphonium (TPP), Szeto-Schiller (SS) peptides and the mitochondria-penetrating peptides (MPPs) were used to direct small molecules to the mitochondria.7 Specifically, the novel peptide antioxidants SS31 and SS20 were used to target the inner mitochondrial membrane and in particular protect the neurons in the MPTP model of PD.6 The mitochondria-targeted antioxidant MitoQ (the TPP-modified ubiquinone) has shown efficacy in both the animal models and human studies of PD.8 The other TPP-modified antioxidants (targeted versions of nitroxide, nitrones, plastoquinons and tocopherol) have also proven effective in animal models of PD.9
Although oxidative stress has been implicated in the pathogenesis of several neurodegenerative diseases, therapies based on exogenous untargeted antioxidants have been disappointing.10 Large quantities of antioxidants are needed to achieve protective effects in the CNS, as most exogenous antioxidants do not efficiently cross the blood–brain barrier owing to their hydrophilic nature. Furthermore, administration of antioxidants is limited due to their toxicity at high doses, resulting in a small therapeutic window for these agents.10 This emphasises the need for alternative strategies to therapeutically counteract the detrimental effects of reactive oxygen species (ROS) and restore the cellular redox balance. A promising candidate to limit ROS-mediated damage is the activation of endogenous antioxidant enzymes present in the CNS, such as catalase, superoxide dismutase, and peroxiredoxins.11
Transcription of these cytoprotective proteins is under control of the nuclear transcription factor NF-E2-related factor 2 (Nrf2), which plays a central role in the regulation of the cellular redox status.12 A variety of the natural Nrf2 activators have proven effective in both in vitro and in vivo models of neurological disorders.13 These activators (such as triterpenoids (TP)) were shown to offer neuroprotection against MPTP-induced toxicity.14 Synthetic TP inhibits oxidative stress and cellular inflammatory processes by potently activating the antioxidant response element (ARE)-Nrf2-Keap1 signalling pathway. Such activation results in a coordinated induction of a battery of cytoprotective genes, including antioxidant and anti-inflammatory genes. It has been proposed that the new activator of the Nrf2 pathway dimethyl fumarate (DMF) may be used for the treatment of not only multiple sclerosis, but PD too. It was recently shown that monomethylfumarate, which is a bioactive metabolite of DMF, offers neuroprotection in the MPTP model of PD.15 This suggests that activation of the Nrf2/ARE signalling pathway might represent a therapeutic target for the treatment of PD.
The novel strategy for mitochondrial pharmacology is connected with using small molecules to modulate systems outside mitochondria that control the number and activity of the organelles. Such modulation can be achieved by altering the transcription of nuclear-encoded mitochondrial proteins that control the major mitochondrial pathways regulating mitochondrial biogenesis; fission, fusion and mitophagy.16 There are now a limited number of compounds that specifically regulate mitochondrial fission and fusion: Mdivi-1 (mitochondrial division inhibitor) that inhibits dynamin-related protein1 (Drp1) and 15-oxospiramilactone (S3) that inhibits USP30, a mitochondrially localised deubiquitinase, and hydrazine (M1) with an unknown target.17 These small molecules may represent useful starting points for drugs that are pro-fusion, ie, they either block fission (Mdivi-1) or enhance fusion (M1, S3).18 Recently, inhibition of Drp1 has been tested in two PD models: genetic (aPINK1–/–mouse) and toxic (the MPTP mouse model).19 The authors demonstrated that: 1) Drp1 inhibition attenuates neurotoxicity and dopamine release deficits in vivo, and 2) pushing the balance of fission-fusion toward fusion is beneficial in PD models with altered mitochondrial function.
It is widely known that PGC1-α is the key transcriptional co-activator protein that upregulates the activity of other transcription factors involved in mitochondrial biogenesis, such as NRF-1 and Transcription Factor A, mitochondrial (TFAM).20 It was established that in mice treated with MPTP to induce Parkinson’s-like phenotypes, PGC1-α overexpression through the neuronal-specific thy-1 promoter significantly protected dopaminergic neurons from MPTP-induced degeneration.21 Helliwell (2016) discussed multiple routes to PGC1-α activation using the low-molecular-weight compounds that target Sirt1, AMPK and PPAR. Resveratrol (a natural stilbenoid found in the skin of grapes) was initially proposed as an activator of Sirt. Resveratrol has been tested in a mouse model of PD where PGC1-α is a likely efficacy target. A recent study showed that necdin, which stabilises PGC-1α, protects against neurotoxicity induced by MPTP.18
It is known that cells have developed a mechanism to eliminate dysfunctional mitochondria via mitophagy. The PINK 1/Parkin-dependent mitophagy has been predominantly implicated in the pathogenesis of PD.22 A novel concept for the treatment of mitochondrial disorders by targeting regulatory components of the mitophagy machinery and upregulating this pathway has been considered now as valuable for PD therapy.22 There are several compounds that increase Parkin function in vitro, including epigenetic agents, cholesterol synthesis modulators, and JNK inhibitors.23 In particular, it has been found that the small molecule JNK inhibitors protect dopaminergic neurons in an MPTP model of PD.24 The activation of a PINK1 neo-substrate (PINK1 yielded kinetin triphosphate) by the kinetin triphosphate precursor (kinetin) administration was able to increase the activity of both wild-type and disease-mutant (PINK1G309D ) directing Parkin to depolarise mitochondria in cellular models of PD.25 It is known that Parkin’s enzymatic functions are antagonised by de-ubiquitination enzymes (DUBs).26 Firstly, small molecule DUB inhibitors as well as Parkin activators are already under development and could furnish the next generation drugs for disease-modifying therapies.4
In our recent study, we have found that use of the new compound Capicor (Meldonium connected with γ-butyrobetain) in PD patients’ therapy results in overexpression of Parkin in leukocytes, normalisation of prooxidant-antioxidant balance in blood plasma, prevention of mitochondrial degradation, stimulation of mitochondrial fusion and elimination of damaged mitochondria in platelets via the mitophagy pathway.27 This compound can act through the DJ-1-connected pathway, which works in parallel with PINK1/Parkin. As has been shown in rotenone- and MPTP-treated mice, phenylbutyrate increased DJ-1 expression, protected DA neurons in SNpc and reduced deterioration in motor and cognitive functions.28
Schapira et al. (2014) proposed several target pathways in PD that merit further study.5 These targets include agents that might improve mitochondrial function or increase degradation of defective mitochondria, kinase inhibitors, calcium channel blockers, and approaches that interfere with the misfolding, templating and transmission of α-synuclein. In this review, we have elucidated the promising pharmacological interventions that modulate different pathways involved in the development of mitochondrial dysfunction and oxidative stress in PD. Such pathways have been implicated in mitochondrial dynamics and clearance dysregulation of mitochondrial biogenesis, fission, fusion, and the mitophagy machinery as well as in molecular mechanisms of antioxidant cell defence.
Biographies
DR OLGA GONCHAR studied for her PhD in the Physiology of Human and Animals at the Bogomolets Institute of Physiology, National Academy of Science, Ukraine. Her research has addressed various methods of analysis, including areas of cellular and molecular biology.
REFERENCES
- Bose A, Beal MF. Mitochondrial dysfunction in Parkinson’s disease. J Neurochem 2016; 139(Suppl.1):216-31.
- Smith RAJ, Hartley RC, Cocheme HM, Murphy MP. Mitochondrial pharmacology. Trends Pharm Sci 2012;33(6):341–52.
- Ryan BJ, Hoek S, Fon EA, Wade-Martins R. Mitochondrial dysfunction and mitophagy in Parkinson’s: From familial to sporadic disease. Trends Biochem Sci 2015; 40:200–10.
- Truban D, Hou Xu, Caulfield TR, Fiesel FC, Springer W. PINK 1, Parkin, and Mitochondrial Quality Control: What can we learn about Parkinson’s disease pathobiology? J Parkinson’s disease 2017;7:13-29.
- Schapira HV, Olanow CW, Greenamyre JT, Bezard E. Slowing of neurodegeneration in Parkinson’s disease and Huntington’s disease: future therapeutic perspectives. The Lancet 2014;384:545-55.
- Milane L, Trivedi M, Singh A, Talekar M, Amiji M. Mitochondrial biology, targets, and drug delivery. J Control Release 2015;207:40-58.
- Szeto HH, SchillerPW. Novel therapies targeting inner mitochondrial membrane – from discovery to clinical development. Pharm Res 2011; 28:2669-79.
- Smith RAJ, MurphyMP. Animal and human studies with the mitochondria-targeted antioxidant MitoQ. Ann NY Acad Sci 2010;1201:96-103.
- Ghosh A, Solesio ME, Prime TA, Logan A, Murphy MP. Neuroprotection by a mitochondria-targeted drug in a Parkinson’s disease model. Free Radic Biol Med 2010;49:1674-84.
- Moosmann B, Behl C. Antioxidants as treatment for neurodegenerative disorders. Expert Opin Invest Drugs 2002;11:1407–35.
- van Muiswinkel FL, Kuiperij HB. The Nrf2-ARE signalling pathway: promising drug target to combat oxidative stress in neurodegenerative disorders. Curr Drug Targets CNS Neurol Disord 2005;4:267–81.
- Nguyen T, Sherratt PJ, Pickett CB. Regulatory mechanisms controlling gene expression mediated by the antioxidant response element. Annu Rev Pharmacol Toxicol 2003;43:233–60.
- Wu KC, McDonald PR, Liu J, Klaassen CD. Screening of natural compounds as activators of the Keap 1-Nrf2 pathway. Planta Med 2013;80:97–104.
- Yang L, Zhao K, Calingasan NY, Luo G, Szeto HH, Beal MF. Mitochondria targeted peptides protect against 1-methyl-4-phenyl-1,2,3,6-tetrahydropyridine neurotoxicity. Antioxid Redox Signal 2009;11:2095–2104.
- Ahuja M, Ammal Kaidery N, Yang L, et al. Distinct Nrf2 signaling mechanisms of fumaric acid esters and their role in neuroprotection against 1-methyl-4-phenyl-1,2,3,6- tetrahydropyridine-induced experimental Parkinson’s-like disease. J Neurosci 2016;36:6332–51.
- Hudson G. The ageing brain, mitochondria and neurodegeneration. In: Reeve AK, Simcox EM, Duchen MR, Turnbull DM, eds. Mitochondrial Dysfunction in Neurodegenerative Disorders. 2nd ed. Springer Int Publishing 2016;59-80.
- 17. Yue W, Chen Z, Liu H, Yan C, Chen M, Feng D, et al. A small natural molecule promotes mitochondrial fusion through inhibition of the deubiquitinase USP30. Nat Publ Gr 2014; 24(4) : 482-96.
- Helliwell SB. Development of treatments and therapies to target mitochondrial dysfunction. In: Reeve AK, Simcox EM, Duchen MR, Turnbull DM, eds. Mitochondrial Dysfunction in Neurodegenerative Disorders. Springer 2nd ed. Int Publishing 2016;349-371.
- 19. Rappold PM, Cui M, Grima JC, Fan RZ, de Mesy-Bentley KL, Chen L, Zhuang X, Bowers WJ, Tieu K. Drp1 inhibition attenuates neurotoxicity and dopamine release deficits in vivo. Commun 2014; 5:5244.
- Ventura-Clapier R, Garnier A, Veksler V. Transcriptional control of mitochondrial biogenesis: the central role of PGC-1alpha. Cardiovasc Res 2008;79:208-17.
- Mudò G, Mäkelä J, Di Liberto V, et al. Transgenic expression and activation of PGC-1α protect dopaminergic neurons in the MPTP mouse model of Parkinson’s disease. Cell Mol Life Sci 2012; 69:1153–65.
- Otten EG, Manni D, Korolchuk VI. Mitochondrial degradation, autophagy and neurodegenerative disease. In: Reeve AK, Simcox EM, Duchen MR, Turnbull DM, eds. Mitochondrial Dysfunction in Neurodegenerative Disorders. 2nd ed. Springer Int Publishing 2016;255-278.
- Hasson SA, Fogel AL, Wang C, MacArthur R, Guha R, Heman-Ackah S, et al. Chemogenomic profiling of endogenous PARK2 expression using a genome-edited coincidence reporter. ACS Chem Biol 2015;10:1188-1197.
- Chambers JW, Pachori A, Howard S, Ganno M, Hansen D, Kamenecka T, et al. Small molecule c-jun-N-terminal kinase inhibitors protect dopaminergic neurons in a model of Parkinson’s disease. ACS Chem Neurosci 2011;2(4):198-206.
- Hertz NT, Berthet A, Sos ML, Thorn KS, Burlingame AL, Nakamura K, Shokat KM. A neo-substrate that amplifies catalytic activity of Parkinson’s-disease-related kinase PINK1. Cell 2013;154:737–47.
- 26. Bingol B, Tea JS, Phu L, Reichelt M, Bakalarski CE, Song Q, Foreman O, Kirkpatrick DS, Sheng M. The mitochondrial deubiquitinase USP30 opposes parkin-mediated mitophagy. Nature 2014;510:370–75.
- 27. Mankovska IM, Rosova KV, Gonchar OO, Nosar VI, Bratus LV, Drevitska TI, Karasevich NV, Karaban IM. Effect of Capicor on the Parkinson’s disease pathogenic links. Fiziol Zh. 2018;64(1):16-24 [Ukraine].
- Inden M, Kitamura Y, Takeuchi H, Yanagida T, Takata K, Kobayashi Y, Taniguchi T, Yoshimoto K, Kaneko M, Okuma Y, Taira T, Ariga H, Shimohama S. Neurodegeneration of mouse nigrostriatal dopaminergic system induced by repeated oral administration of rotenone is prevented by 4-phenylbutyrate, a chemical chaperone. J Neurochem 2007;101:1491–1504.
The rest of this content is restricted - login or subscribe free to access
Why subscribe? Join our growing community of thousands of industry professionals and gain access to:
- quarterly issues in print and/or digital format
- case studies, whitepapers, webinars and industry-leading content
- breaking news and features
- our extensive online archive of thousands of articles and years of past issues
- ...And it's all free!
Click here to Subscribe today Login here