Upstream cell culture processes for the production of therapeutic proteins
Posted: 31 July 2018 | Jonathan P. Welsh | No comments yet
The upstream process encompasses the initial transfections of a gene of interest into host cells, the cloning of cell lines with desirable characteristics (e.g. high productivity) and subsequent scale-up to large scale manufacture.
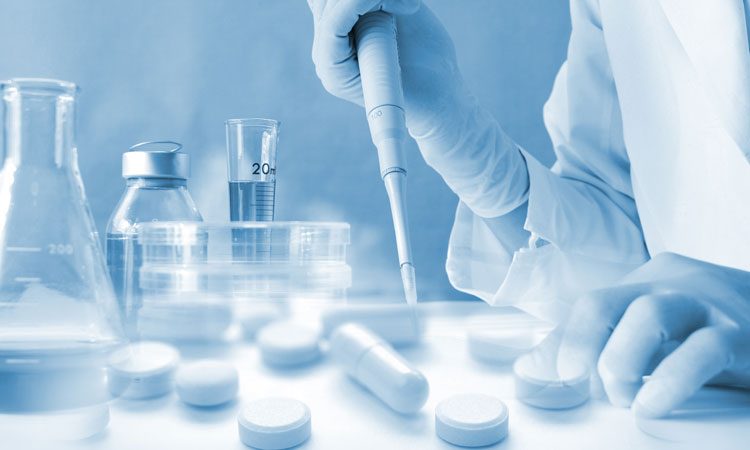
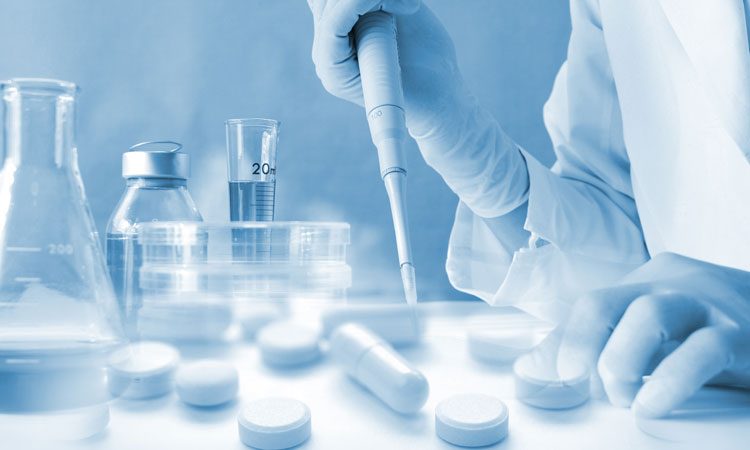
Jonathan P. Welsh, Principal Scientist, Centre for Process Innovation, National Biologics Manufacturing Centre, Darlington, UK
In 2017, seven of the top 10 drugs in terms of market value were of biological origin, including the top seller Humira (adalimumab) with sales of $18.4 billon.1 They are the backbone of the drug discovery pipelines of many major pharmaceutical companies. These biologic therapeutics can be defined as proteins derived from eukaryotic cell culture processes. The ability of eukaryotic cells to undertake complex post translational modifications makes them the vehicles for manufacturing proteins emerging from development pipelines.
The upstream process encompasses the initial transfections of a gene of interest into host cells, the cloning of cell lines with desirable characteristics (e.g. high productivity) and subsequent scale-up to large scale manufacture.
Cell line development
Following the initial drug discovery with the identification and design of efficacious molecules, the manufacturing considerations are brought to the fore. Initially, transient transfections may give sufficient material for early assessment of product characteristics and efficacy. However, ICH guidelines stipulate that a therapy must be derived from a clonal cell line, as a way of ensuring consistency.2 The host cell of choice is usually a derivative of the CHO cell. Most manufacturers and biopharmaceutical companies now have their own proprietary host cells, tested to be suitable for current good manufacturing processes (cGMP). The plasmid vector with the gene for the therapeutic protein is transfected into the host cell, usually by electroporation. There are technologies that allow the gene to be inserted into a region of the genome where expression will not be silenced by epigenetic factors.3 Following transfection, selection pressure can be exerted on the cells to allow the survival of those transfected with the gene of interest. For example, in CHO cells with the glutamine synthase gene knocked out (CHO GS-/-), if transfected with a plasmid containing the GS gene to make endogenous glutamine, simply culturing in medium without added glutamine will provide the selection pressure. Once pools of expressing cells have been established, cells are cloned out by methods such as fluorescent activated cell sorting (FACS) or more recently single-cell printers.4) The number of clones established can be up to several hundred to allow a suitable number to be screened. Usually an imaging technique is used to observe colonies emerge from single points.5 These data satisfy the regulators that cell lines progressed are from single clones. At this stage, the colonies can only be assessed for productivity due to the small amounts of material available, but it ensures that the highest producing clones are progressed. Minibioreactors that provide accurate scale-down models of the production process have been game changers in the past decade. The best known examples are systems such as the ambr15 and 250 (TAP/Sartorius).6 In these systems the production process, usually fed-batch can be run, and the top performing clones progressed. At this stage, sufficient material is generated to allow for some product characteristics to be assayed. These may include SDS-PAGE for molecular heterogeneity, iCEF for charge variants, glycosylation patterns and the amount of product aggregation. Any challenging liabilities in the product can be discovered at this stage. Research banks of the top clones are then made. They can then be further assessed in lab-scale bioreactors which also provide a feed stream for downstream processing. Finally stability studies are performed to look for the consistency of expression and product quality in the manufacturing window. This process can generate a cGMP banked cell line in 12-14 weeks.
Process optimisation
Following the establishment of a cell line, the protein production process is assessed for robustness and consistency. This stage is crucial is ascertaining that the drug product matches the molecule that emerged from the drug discovery process. Many manufacturers have established platform processes. This has an advantage of fitting with the facility and having personnel suitably trained and experienced in operating the process. The disadvantage is that it may not be appropriate for every product type. As each cell line may behave differently in the platform, and different molecules may be more challenging to produce, an optimisation step is routinely employed. Again minibioreactor systems are now routinely used for this (Figure 1). They allow a design of experiment (DoE) approach to be used. Risk assessments can be undertaken to establish the variables that will most affect the process integrity and which are most likely to deviate during manufacturing. The process is run at the edge of limits with outputs such as cell growth, productivity and product quality. A design space for running the process established and within that an operating space defined. When transferred into the cGMP environment if the process is run within the operating space a consistent product quality should be maintained.
Production Platforms
Moving into cGMP production, where a locked down process, developed in the research lab is used for the manufacture of the active pharmaceutical. A process can be evaluated at pilot scale (between 50-500L), which may also provide representative material for pre-clinical toxicology tests and then progressed to production scale (between 1,000 -20,000L) for cell culture.7
The most common approach is a fed-batch system using stirred-tank bioreactors. The scale-up from lab-scale to pilot and production is well established for this mode of operation. Typically a batch will run for around 14 days following inoculation and then harvested. It is challenging for new approaches to break into the space, even if they have advantages e.g. simplicity and lower cost. Economic and practical reasons may be a driver for change, particularly if more batches in smaller volumes are needed.
Continuous processing is currently enjoying a lot of interest. Bioprocessing is one of the few industries where continuous processes are not employed as commonly as in other industries. The use of continuous processing as a way of ensuring a more consistent product is something that has won favour. In these processes, the product in solution is continuously removed from the vessel, replaced with fresh medium and the biomass retained, sometimes reaching very high densities.8
There are hybrid approaches better suited to existing plant and processes. For example using a continuous culture in the seed train, allows a higher cell number to be generated to inoculate the production vessel, which although still operated as a fed batch will give the same productivity over a shorter time period.9
Traditionally, stainless-steel plants have been used for upstream manufacture. These have a high capital cost and require validated and time consuming cleaning between batches, even more so if the same plant is used for multiple products. The trend for single use technologies has alleviated this, although the issues with leachables and extractables due to the film composition are still of some concern.
Future trends
What is exciting about the field is that so much is not known and yet to be discovered. Integrated teams of molecular biologists, process engineers and data analysts are taking on the new challenges.
If more products for different patient cohorts are needed in the future, there will have to be a more parallel approach to cell line development. The emergence of systems such as Berkeley Lights™ are providing the automation and parallel processing power required.
The CHO genome has been sequenced, and comparative studies to understand differential gene expression of high and low producing cell lines.10 Advances in gene editing approaches using CRISPR-Cas9 for example, means cell lines can be edited more readily. In the future, it might become more common to establish host cells for particular product types rather than the one-size-fits all approach. As we understand more about the underlying biology and what makes a minimal cell, there will be synthetic biology avenues to explore.
The production of monoclonal antibodies is well established, and titres of 5-10g/L are now possible. The next challenges will be to replicate these feats for an increasingly diverse range of products. These include bi- and tri-specific antibodies and antibody fragments that may have the same efficacy as more complex molecules, but simpler to formulate and store. There are solutions to making these more difficult-to-express molecules.11
Temperature, pH and dissolved oxygen and gas flows into bioreactors were historically the only online measurements made. Metabolites and cell numbers have to be measured using offline methods. This inevitably means that vessels have to be sampled which breaks containment barriers and risks contaminations. However, online methods are available with cell numbers monitored using capacitance probes, and Raman or NIR probes used to monitor key metabolites.12 Currently, these techniques are expensive to implement, especially in the early stages of development using minibioreactor systems. However, this process analytical technology (PAT) will lead to better process understanding and control. An increasing desire to capture and digitalise the data generated in a process will improve predictive models of culture performance.
There has interest in using microbial systems for the production of more complex proteins. In a recent report.13 The mAb yield using the fungus Myceliophthora thermophilia (Dyadic C1) platform was 2.4 g/l/day. A reported 9 g/l of the target mAb in a 90 hour fermentation.
Drug discovery scientists continue to uncover ever more diverse molecules. The upstream cell culture process is pivotal to the quality of the products produced. The challenge for the bioprocessing community is to turn these discoveries into safe and effective therapies consistently, to treat disease and improve or maintain quality of life.
Biography
Jonathan P. Welsh
Jonathan obtained his PhD at the University of Birmingham, UK. He gained experience in upstream cell culture at Lonza Biologics, with cell line constructions, bioreactor operations, process optimisations and process transfers. He joined the Centre of Process Innovation in 2014 to help establish their upstream capabilities for process development. Currently his interests are in understanding the causes of variation in cell culture processes, through the application of PAT and advanced data analysis.
Related topics
Biopharmaceuticals, Biotherapeutics, Protein