Investigation of venom use in stem cell differentiation
Posted: 16 June 2015 | Ginika J Jideofor (Cranfield Institute of Health), Jeff D. Newman (Cranfield Institute of Health) | No comments yet
Stem cells differentiate to form more than 200 specialised cells, including erythroid bodies, haematopoietic cells, myogenic cells, neural cells, signalling cells and structural cells, such as hepatocytes. Differentiation of stem cells triggers a number of variable changes in the cell, such as membrane potential, responsiveness to signal, metabolic activities, and most importantly their specialisation often involving size and shape…
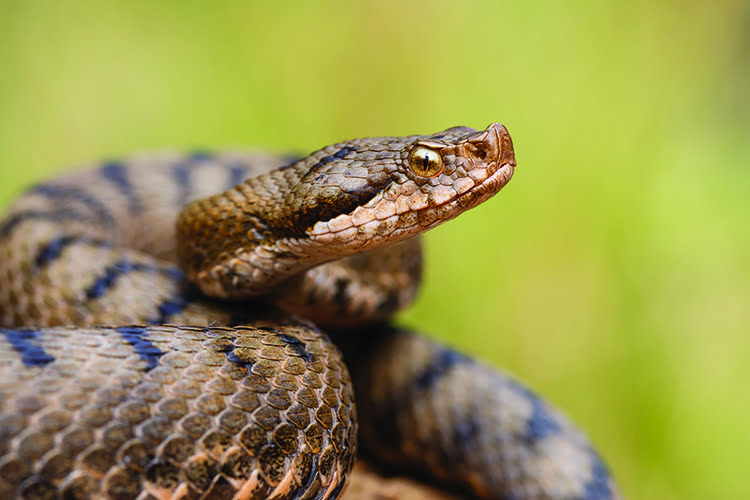
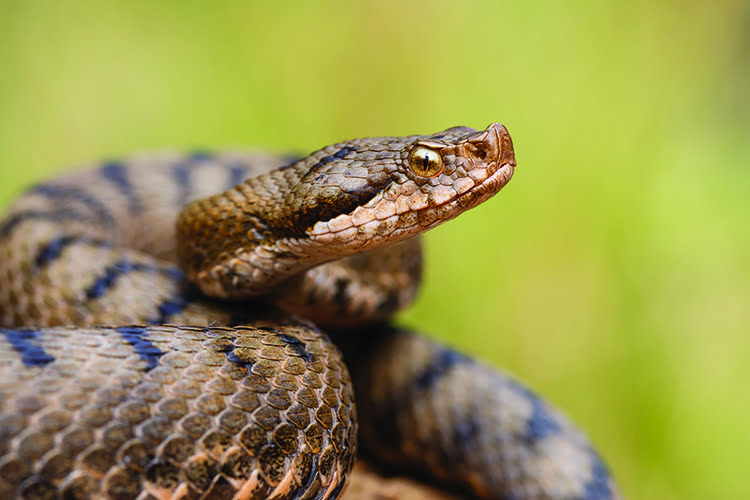
Alterations in gene expression make a very significant contribution to these changes and continue through the life of the differentiated cell. The DNA sequence usually remains the same during cellular differentiation, with a few exceptions (as with germ cells). Stem cell differentiation is crucial in embryogenesis; numerous specific cells are produced in precise locations to form the organism in a carefully controlled sequence. Embryonic stem (ES) cells formed during this process are being investigated to treat a wide range of diseases.
The success of the clinical application of ES cells depends on proper isolation, manipulation, expansion and differentiation of the cells1. The identification of the appropriate growth factors necessary to maintain the proliferation of undifferentiated state of ES cell is important in the development of the optimal culture condition. In addition, the efficient control of the in-vitro differentiation of the ES cell to a specific cell type is a crucial issue in ES cell research.
Variable ES cell differentiation methods have been developed for certain tissues. The frequently used method is the aggregation of ES cells creating embryoid bodies (EB). The stem cells are cultured in the absence of differentiating signals, thus, the ES cells proliferate into specialised cells in the form of these embroid bodies. The cells induce each other to differentiate, which is similar to early embryogenesis. In human ES cells, EBs are generated and then isolation of the ES cell colonies takes place using either mechanical transfer or enzymatic transfer from the EBs. The selected colonies are grown in different media and plates depending on the pathway required.
ES cells can also be differentiated by combined culturing with specific feeder cells, which is usually employed in producing haematopoietic and neuronal stem cells from ES cells. This method is highly effective in programming ES cells into a specific lineage. However, the separation of feeder cells from specialised cells is critical because the remains of the feeder cells pose concern due to xeno-contamination. ES cell differentiation in a specific monolayer substrate without feeder cells reduces the influences of other factors that may affect differentiation2. The key limiting step has been finding the parameters and reagents to initiate and maintain the differentiated phenotype.
Signalling pathways
The following are examples of signalling pathways involved in stem cell differentiation.
Hedgehog (Hh) signalling pathway
This pathway is crucial in embryonic development across a vast phylogenetic distance. The hedgehog signalling protein is expressed in concentration gradients in various parts of the embryo to coordinate development of multicellular organisms. Among the three hedgehog homologues, sonic is the most researched. This pathway is a highly coordinated and orchestrated network3. When sonic 29 hedgehog (shh) protein is present, its protein complex will release a complete Gli3 in the nucleus to stimulate Hh target genes such as Gli1. Current research suggests that incorrect activation of Hh pathway may contribute to human tumour formation. Most tumours investigated such as skin, brain and muscles have been demonstrated to show Hh pathway activation. Therefore, tight control of such a pathway is needed to produce safe stem cell therapies.
Hippo signalling pathway
Like Hh this is another evolutionary conserved pathway involved in regulation of organ size in an animal by controlling proliferation and apoptosis. Hippo signalling occurs through a kinase-dependant mechanism to control transcription of sensitive genes.
Wnt (wingless-type) signalling pathway
This plays a major role in the development of organs and tissues including cell fate determination, axis and polarity of cells and tissues. Wnt signalling has also been implicated in stem cell renewal and expression of wnt is under very tight regulation during embryogenesis.
Protein kinase receptor
The combined effect of growth factors and cytokines with haematopoietic stem cells determines the possibility of differentiation into a specific lineage or self-renewal of the stem cell depending on the stress level. Proliferation and apoptosis of stem cells are regulated by activated protein kinase receptors through phosphorylation of eukaryotic initiation factor5. There is a suggestion that the loss of protein kinase receptor expression is associated with increased growth and malignancy.
A powerful bite
Venoms are complex mixtures made up of carbohydrates, peptides, proteins, nucleotides, ions and biogenic amines, with a small amount of lipids and amino acids. Venom is a toxin injected into victims by venomous animals such as snakes, spiders, scorpions, insects, fish, jellyfish and others through bites or stings. Their complexity varies from ordinary phenols and organic acids in some venom, to sophisticated combinations of proteins with a wide range of pharmacologically active substances, as often found in snake venoms.
Many venom components have significant medical importance. To give some examples, apamin is a component of honey bee venom, which efficiently blocks the SK2 and SK2 genes expression in an animal model of atherosclerosis, and has been shown to exert antifibrosis effects6, while a snake venom ‘thrombin-like enzyme’ known as batroxobin, derived from Bothrops atrox moojeni, is currently used in the treatment of deep vein thrombosis through thrombolysis. It continues to degrade the fibrin chains even after the clot has formed. Another example of a venom component is C-type lectin, which is a non-enzymatic, calcium dependent carbohydrate-binding protein. It has been utilised in cancer therapy, through reduction in cell viability of gastric carcinoma cells due to the cytotoxic properties disrupting the actin filaments and apoptosis. Cyclin D is involved in controlling the progression of cell cycle by activating cyclin-dependent kinase (Cdk) enzyme. Cyclin-D1 amplification has been a point of discussion in cancer therapy resistance using tamoxifen.
Disintegrins are a family of proteins found in viper venom that interfere with integrin signalling pathways. These affect many cell adhesion pathways including the coagulation pathway, by inhibiting the aggregation of platelets7. In total approximately fifteen drugs on the market are known to have involved venom in their research or are venom peptides.
So can venoms be used to modulate stem cell differentiation? In order to answer this question, a project undertaken looked at a representative group of stem cell markers in two pathways where venoms are known to act; haematopoiesis (blood cell differentiation) and neuroopoiesis (nerve cell differentiation). Approximately 140 stem cell markers were compiled from the published literature, of which 17 have a connection with venom components (Table 1).
The improved understanding of stem cell pathways and their application as therapeutic agents is the basis of hope for many of incurable diseases. Stem cells have the potential to regenerate and repair tissue injury or aid in disease recovery. This regenerative ability of stem cells applied in certain disorders, such as diabetes, stroke, spinal cord injury, macular degeneration, burns, rheumatoid arthritis, heart disease and osteoarthritis, will promote longevity.
The aim of this study was to investigate weather venom peptides could be screened as novel cheaper ways to differentiate stem cells. The table was produced through critically reviewing the literature, listing the stem cell markers in these pathways and then searching for overlap with venom publications. The result has only scratched the surface but suggests that venom components such as snake venom fibrin sealant, scorpion venom peptides and batroxobin could have a role in stem cell research. Potentially screening venom peptides could elucidate unknown pathways and lead to further advances in stem cell biology.
(Table 1: Stem cell markers with their venom connection)
Protein |
Description |
Venom connection |
Venomous species |
Venom protein involved |
Alkaline phosphatase (AP) |
Stem cell differentiation marker8 |
Histochemically detected in the venom apparatus |
Endoparasioid wasp (Pteromalus puparum)9 |
Alkaline phosphatase |
CD9 (member of tetraspanin superfamily) |
Contributes to regulating physiological processes 10 |
Fertilin β (ADAM2), whose disintegrin domains are homologous to integrin ligands in snake venoms11 |
Viper |
Integrin |
Protein kinase G (PKG) |
Modulates vascular smooth muscle cells’ phenotype12, 13 |
Dendroaspis natriuretic peptide inhibits the cardiac L-type Ca2+ channel activity through PKG activation14 |
Mamba snake (Dendroaspis angusticeps) |
Dendroaspis natriuretic peptide |
Basic fibroblast growth factor (bFGF) |
Used as feeder free media2 |
Disintegrins and C-type lectin-like proteins which inhibit integrin-ligand interactions, thereby inhibiting the activation and differential expression of angiogenic factors15 |
Snakes (Vipera xantina palestinae, Calloselasma rhodostoma, Echis multisquamatus) |
Vixapatin (VP12), usually known as C-type lectin-like proteins |
Transforming growth factor-beta (TGFβ1) |
Essential in self-renewal activity2 |
Like barbourin, mouse and human pregnancy-specific glycoproteins (PSGs), binds to αIIbβ3 and inhibits the platelet fibrinogen interaction |
Pygmy rattlesnake (S. m. barbouri) |
Alpha-ketoglutarate decarboxylase, a platelet integrin αIIbβ3 antagonist |
Transforming growth factor-alpha (TGF-α) |
Transmembrane precursor which exists on cell surface16 |
Melittin modulates multiple cellular functions17 |
Bee venom |
Melittin |
E-cadherin |
Participates in embroid bodies formation2 |
Melittin modulates multiple cellular functions17 |
Bee venom |
Melittin |
|
|
Change index such as higher E-cadherin and lower N-cadherin18 |
Not mentioned |
Snake venom cystatin (sv-cystatin) |
β-catenin |
Present in apical domain of embryonic neuroepithelial cells19 |
The administration of P. Nigriventer venom in the hippocampus of rats shows changes in the expression of VEGF, F1t-1, F1k-120 |
Spider (Phoneutria nigriventer) |
Ion channel blocking peptides |
Cyclin D1 |
Glucagon-like peptide receptor |
Cyclin D1 and c-Myc, determinants of cell proliferation, are up-regulated by Exd421 |
Gila monster (Heloderma suspectum) |
Exendin -4 |
N-cadherin |
Present in apical domain of embryonic neuroepithelial cells19 |
Change index such as higher E-cadherin and lower N-cadherin18 |
Puff adder (Bitis areitans) |
Snake venom cystatin (sv-cystatin) |
Glial fibrillary acidic protein (GFAP) |
GFAP is a biomarker found in CNS22 |
Ventral root avulsion repaired with snake venom fibrin sealant at the point of detachment contributed to neuroprotection and preservation of the synaptic network at the micro environment of the lesioned motoneurons23 |
Snake venom |
Fibrin sealant |
Aquaporin-4 and GFAP |
GFAP is a biomarker found in the CNS22 |
P. nigriventer venom increases the expression of aquaporin-4 (AQP4) and GFAP24 |
Brazilian armed spider (Phoneutria nigriventer) |
P. nigriventer venom |
Phosphodiesterase 5 (PDE5) |
Inhibition of phosphodiesterase 5 stimulates neural stem cell proliferation13 |
PDE5 stimulates proliferation by inhibiting the hydrolysis of cGMP |
Pit viper (Trimeresurus stejnegeri) |
Phosphodiesterase from Trimeresurus stejnegeri venom (TS-PDE)25 |
Cyclin-dependent kinase inhibitor protein (p27kip1) |
p27 Kip1 regulates cell cycle and decision of adult neural stem cells fate13,26 |
Tumour cell lines maintained in culture when exposed to psalmotoxin-1 (PcTX-1) leads to accumulation of p21Cip1 and p27Kip1 which slows proliferation and decreases phosphorylation of ERK1/227 |
West Indies tarantula (Psalmopoeus cambridgei) |
Psalmotoxin-1 (PcTX-1) |
GLP-1 |
Long-acting agonist for gut-derived insulinotropic hormone GLP-121 |
Exd4 positively regulates proproliferative genes, cyclin D1 and c-myc through the Wnt signalling pathway21 |
Gila monster (Heloderma suspectum) |
Exendin-4 (Exd4) |
VE (vascular endothelial)-Cadherin |
Early endothelial marker 28 |
Loss of cell anchorage and cell-to-cell adhesion following venom exposure was accompanied by changes in the distribution of the α₅β₁ integrin and VE-cadherin |
Brown spider (Loxosceles sp.)29 |
Unknown; possibly sphingomyelinase |
CD34 |
A marker utilised in identification and purification of satellite cells and skeletal muscle regeneration30 |
Batroxobin increases the levels of CD34+, CD31+, CD34+/CD31+ and vascular endothelial cadherin cells in patients’ peripheral blood after seven days of treatment31 |
Pit viper (Bothrops atrox) |
Batroxobin, a thrombin-like enzyme |
IL-3 |
Regulates the expression of certain oncogenes and protects against radiation-induced apoptosis on bone marrow cells32 |
Scorpion venom peptide SVPII increases the expression of IL-3 receptor and promotes proliferation of irradiated cells32 |
Scorpion (Buthus Martti Karsch) |
Scorpion venom peptide II |
Stem cell factor |
A ligand of c-kit, a hematopoietic growth factor33 |
Haematopoietic recovery after radiation was probably due to a mechanism of stimulating the cytokine release by the Scorpion venom peptide34 |
Scorpion (Buthus martensii Karsch) |
Scorpion venom peptide II |
Fms-like tyrosine kinase 1 (Flt-1) |
‘Soluble VEGFR1 is a promising biomarker and therapeutic target for angiogenesis diseases’35, 36 |
F1t-1 and Flk-1 exhibited nuclear translocation, thereby probably possess the potential mechanism for regulating VEGF |
Spider (Phoneutria nigriventer)20 |
Phoneutria nigriventer spider venom |
Foetal liver kinase 1/kinase insert domain receptor/VEGFR2 |
‘VEGFR2 is the dominant mediator of proangiogenesis’35, 20, 36 |
F1t-1 and Flk-1 exhibited nuclear translocation, thereby probably possess the potential mechanism for regulating VEGF |
Spider (Phoneutria nigriventer)20 |
Phoneutria nigriventer spider venom |
Conclusion
The improved understanding of stem cells and their application as therapeutic agents is the basis of hope for the development of better therapy for numerous diseases. Stem cells have the potential to regenerate and repair tissue injury or aid in disease recovery in disorders such as diabetes, stroke, spinal cord injury, macular degeneration, burns, rheumatoid arthritis, heart disease and osteoarthritis. One of the hurdles is the difficulty and cost of promoting and controlling stem cell differentiation. Several venom components, including snake venom fibrin sealant, scorpion venom peptides and batroxobin could have potential for use in this field. This project has just scratched the surface of how looking to nature, specifically venoms could help unlock problems in stem cell research due to the potent pharmacological action of venom components.
Biographies
References
- Strulovici, Y., Leopold, P. L., O’Connor, T. P., Pergolizzi, R. G. and Crystal, R. G. (2007), “Human embryonic stem cells and gene therapy”, Molecular Therapy, vol. 15, no. 5, pp. 850-866.
- Moon, S. Y., Park, Y. B., Kim, D., Oh, S. K. and Kim, D. (2006), “Generation, culture, and differentiation of human embryonic stem cells for therapeutic applications”, Molecular Therapy, vol. 13, no. 1, pp. 5-14.
- Kubo, M., Nakamura, M., Tasaki, A., Yamanaka, N., Nakashima, H., Nomura, M., Kuroki, S. and Katano, M. (2004), “Hedgehog signaling pathway is a new therapeutic target for patients with breast cancer”, Cancer research, vol. 64, no. 17, pp. 6071-6074.
- Yokoyama, N. N., Shao, S., Hoang, B. H., Mercola, D. and Zi, X. (2014), “Wnt signaling in castration-resistant prostate cancer: implications for therapy”, Am J Clin Exp Urol, vol. 2, no. 1, pp. 27-44.
- Liu, X., Bennett, R. L., Cheng, X., Byrne, M., Reinhard, M. K. and May, W. S.,Jr (2013), “PKR regulates proliferation, differentiation, and survival of murine hematopoietic stem/progenitor cells”, Blood, vol. 121, no. 17, pp. 3364-3374.
- Lee, W., Kim, K., An, H., Kim, J., Lee, S., Han, S., Pak, S. C. and Park, K. (2014), “Apamin inhibits hepatic fibrosis through suppression of transforming growth factor β1-induced hepatocyte epithelial-mesenchymal transition”, Biochemical and biophysical research communications.
- Calvete, J. J., Marcinkiewicz, C., Monleón, D., Esteve, V., Celda, B., Juárez, P. and Sanz, L. (2005), “Snake venom disintegrins: evolution of structure and function”, Toxicon, vol. 45, no. 8, pp. 1063-1074.
- Ye, G., Li, C., Xiang, X., Chen, C., Zhang, R., Yang, X., Yu, X., Wang, J., Wang, L. and Shi, Q. (2014), “Bone Morphogenetic Protein-9 Induces PDLSCs Osteogenic Differentiation through the ERK and p38 Signal Pathways”, International Journal of Medical Sciences, vol. 11, no. 10, pp. 1065-1072.
- Zhu, J. Y., Yin Ye, G., Fang, Q. and Hu, C. (2010), “Alkaline phosphatase from venom of the endoparasitoid wasp, Pteromalus puparum”, Journal of insect science (Online), vol. 10, pp. 14.
- Okamoto, T., Iwata, S., Yamazaki, H., Hatano, R., Komiya, E., Dang, N. H., Ohnuma, K. and Morimoto, C. (2014), “CD9 negatively regulates CD26 expression and inhibits CD26-mediated enhancement of invasive potential of malignant mesothelioma cells”, PloS one, vol. 9, no. 1, pp. e86671.
- Zhu, X. and Evans, J. P. (2002), “Analysis of the roles of RGD-binding integrins, alpha(4)/alpha(9) integrins, alpha(6) integrins, and CD9 in the interaction of the fertilin beta (ADAM2) disintegrin domain with the mouse egg membrane”, Biology of reproduction, vol. 66, no. 4, pp. 1193-1202.
- Yang, H., Kim, B., Kim, J., Kwon, Y., Jin,S., Lee, J., Cho, H., Lee, H., Kang, H. and Oh, B. (2013), “PPARγ modulates vascular smooth muscle cell phenotype via a protein kinase G-dependent pathway and reduces neointimal hyperplasia after vascular injury”, Experimental & molecular medicine, vol. 45, no. 11, pp. e65.
- Santos, A. I., Carreira, B. P., Nobre, R. J., Carvalho, C. M. and Araújo, I. M. (2014), “Stimulation of neural stem cell proliferation by inhibition of phosphodiesterase 5”, Stem cells international, vol. 2014.
- Park, S., Kim, T., Han, M., Ha, K., Kim, S. and Kwak, Y. (2012), “Dendroaspis natriuretic peptide regulates the cardiac L-type Ca2 channel activity by the phosphorylation of α1c proteins”, Experimental & molecular medicine, vol. 44, no. 6, pp. 363-368.
- Momic, T., Cohen, G., Reich, R., Arlinghaus, F. T., Eble, J. A., Marcinkiewicz, C. and Lazarovici, P. (2012), “Vixapatin (VP12), a c-type lectin-protein from Vipera xantina palestinae venom: characterization as a novel anti-angiogenic compound”, Toxins, vol. 4, no. 10, pp. 862-877.
- Vassar, R. and Fuchs, E. (1991), “Transgenic mice provide new insights into the role of TGF-alpha during epidermal development and differentiation”, Genes & development, vol. 5, no. 5, pp. 714-727.
- Sommer, A., Fries, A., Cornelsen, I., Speck, N., Koch-Nolte, F., Gimpl, G., Andra, J., Bhakdi, S. and Reiss, K. (2012), “Melittin modulates keratinocyte function through P2 receptor-dependent ADAM activation”, The Journal of biological chemistry, vol. 287, no. 28, pp. 23678-23689.
- Tang, N., Xie, Q., Wang, X., Li, X., Chen, Y., Lin, X. and Lin, J. (2011), “Inhibition of invasion and metastasis of MHCC97H cells by expression of snake venom cystatin through reduction of proteinases activity and epithelial-mesenchymal transition”, Archives of Pharmacal Research, vol. 34, no. 5, pp. 781-789.
- Abranches, E., Silva, M., Pradier, L., Schulz, H., Hummel, O., Henrique, D. and Bekman, E. (2009), “Neural differentiation of embryonic stem cells in vitro: a road map to neurogenesis in the embryo”, PLoS One, vol. 4, no. 7, pp. e6286.
- Mendonça, M. C., Soares, E. S., Stávale, L. M., Rapôso, C., Coope, A., Kalapothakis, E. and da Cruz-Höfling, M. A. (2013), “Expression of VEGF and Flk-1 and Flt-1 receptors during blood-brain barrier (BBB) impairment following Phoneutria nigriventer spider venom exposure”, Toxins, vol. 5, no. 12, pp. 2572-2588.
- Liu, Z. and Habener, J.F. (2008). “Glucagon-like peptide-1 activation of TCF7L2-dependent Wnt signaling enhances pancreatic beta cell proliferation” J.Biol.Chem. Volume: 283 Issue: 13 Pages: 8723-8735
- Cikriklar, H. I., Ekici, M. A., Ozbek, Z., Cosan, D. T., Baydemir, C. and Yurumez, Y. (2013), “Investigation of the course of GFAP in blood in the initial 24 hours in rats subjected to minor head trauma”, European review for medical and pharmacological sciences, vol. 17, no. 24, pp. 3391-3397.
- Barbizan, R., Castro, M. V., Rodrigues, A. C., Barraviera, B., Ferreira, R. S. and Oliveira, A. L. (2013), “Motor Recovery and Synaptic Preservation after Ventral Root Avulsion and Repair with a Fibrin Sealant Derived from Snake Venom”, PloS one, vol. 8, no. 5, pp. e63260.
- Stavale, L. M., Soares, E. S., Mendonça, M. C., Irazusta, S. P. and da Cruz Höfling, Maria Alice (2013), “Temporal relationship between aquaporin-4 and glial fibrillary acidic protein in cerebellum of neonate and adult rats administered a BBB disrupting spider venom”, Toxicon, vol. 66, pp. 37-46.
- Peng, L., Xu, X., Guo, M., Yan, X., Wang, S., Gao, S. and Zhu, S. (2013), “Effects of metal ions and disulfide bonds on the activity of phosphodiesterase from Trimeresurus stejnegeri venom”, Metallomics, vol. 5, no. 7, pp. 920-927.
- Gil-Perotin, S., Haines, J.D., Kaur, J., Marin-Husstege, M., Spinetta, M.J., Kim, K.H., Duran-Moreno, M., Schallert, T., Zindy, F., Roussel, M.F., Garcia-Verdugo, J.M. and Casaccia, P. (2011). “Roles of p53 and p27(Kip1) in the regulation of neurogenesis in the murine adult subventricular zone”. European Journal of Neuroscience, Volume: 34 Issue: 7 Pages: 1040-1052
- Rooj, A. K., McNicholas, C. M., Bartoszewski, R., Bebok, Z., Benos, D. J. and Fuller, C. M. (2012), “Glioma-specific cation conductance regulates migration and cell cycle progression”, The Journal of biological chemistry, vol. 287, no. 6, pp. 4053-4065.
- Pavlov, N., Frendo, J., Guibourdenche, J., Degrelle, S. A., Evain-Brion, D. and Badet, J. (2014), “Angiogenin Expression during Early Human Placental Development; Association with Blood Vessel Formation”, BioMed research international, vol. 2014.
- Nowatzki, J., Sene, R. V., Paludo, K. S., Rizzo, L. E., Souza-Fonseca-Guimarães, F., Veiga, S. S., Nader, H. B., Franco, C. R. C. and Trindade, E. S. (2012), “Brown spider (Loxosceles intermedia) venom triggers endothelial cells death by anoikis”, Toxicon, vol. 60, no. 3, pp. 396-405.
- Alfaro, L. A. S., Dick, S. A., Siegel, A. L., Anonuevo, A. S., McNagny, K. M., Megeney, L. A., Cornelison, D. D. W. and Rossi, F. M. V. (2011), “CD34 Promotes Satellite Cell Motility and Entry into Proliferation to Facilitate Efficient Skeletal Muscle Regeneration”, Stem cells, vol. 29, no. 12, pp. 2030-2041.
- Zhang, L., Lu, S.H., Li, L., Tao, Y.G., Wan, Y.L., Senga, H., Yang, R. and Han, Z.C. (2011). “Batroxobin Mobilizes Circulating Endothelial Progenitor Cells in Patients With Deep Vein Thrombosis”. Clinical and Applied Thrombosis-Hemostasis. Volume: 17 Issue: 1 Pages: 75-79.
- Qiu, Y., Jiang, L., Wang, C., Wang, Y., Li, T., Xing, B., Zhou, M., Kong, T. and Dong, W. (2013), “Scorpion venom peptide SPVII promotes irradiated cells proliferation and increases the expression of the IL-3 receptor”, Cell & bioscience, vol. 3, no. 1, pp. 28-3701-3-28.
- Wang, X., Ren, H., Zhao, T., Chen, J., Sun, W., Sun, Y., Ma, W., Wang, J., Gao, C., Gao, S., Lang, M., Jia, L. and Hao, J. (2014), “Stem cell factor is a novel independent prognostic biomarker for hepatocellular carcinoma after curative resection”, Carcinogenesis, vol. 35, issue 10, 2283-2290.
- Dong, W., Wang, L., Kong, T. and He, Y. (2009), “Scorpion venom peptides accelerate hematopoietic recovery of myelosuppression in irradiated mice”, The American Journal of Chinese Medicine, vol. 37, no. 04, pp. 701-712.
- Ema, M., Yokomizo, T., Wakamatsu, A., Terunuma, T., Yamamoto, M. and Takahashi, S. (2006), “Primitive erythropoiesis from mesodermal precursors expressing VE-cadherin, PECAM-1, Tie2, endoglin, and CD34 in the mouse embryo”, Blood, vol. 108, no. 13, pp. 4018-4024.
- Xia, L., Dong, Z., Zhang, Y., Zhang, X., Song, X., Sun, M., Hu, Y., Liu, S., Wang, K. and Qu, X. (2014), “Interleukin-4 and granulocyte-macrophage colony-stimulating factor mediates the upregulation of soluble vascular endothelial growth factor receptor-1 in RAW264. 7 cells—a process in which p38 mitogen-activated protein kinase signaling has an important role”, Journal of Microbiology, Immunology and Infection. Published on-line
Related topics
Disease research, DNA, In Vitro, Kinases, Protein, Stem Cells, Therapeutics