Telomerase – a universal cancer target for immunotherapeutic vaccines
Posted: 23 September 2021 | Dr Jens Bjørheim (Ultimovacs) | No comments yet
In this article, Dr Jens Bjørheim, Chief Medical Officer of Ultimovacs, explains why vaccination has proved the best strategy to target human telomerase and why this complex may be an effective approach to combat cancer.
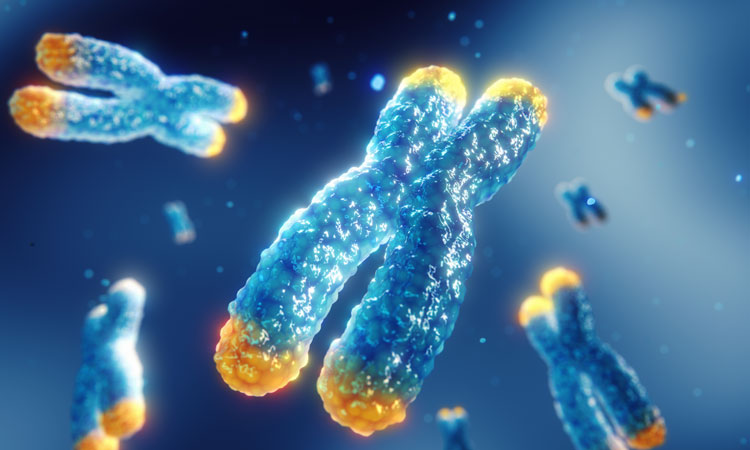
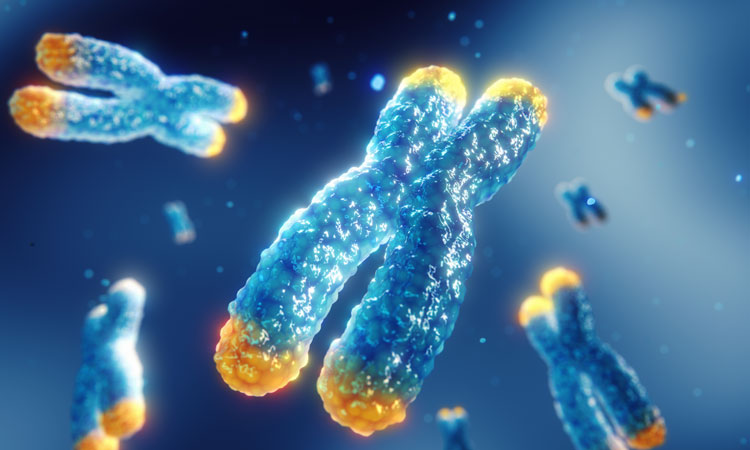
Since the discovery of telomerase in 1994,1 the enzyme has been viewed as a highly attractive target for the development of potential cancer therapeutics. It is expressed broadly across cancer types, fulfils a function essential in rapidly growing cells and is largely quiescent in most normal human tissues. However, small molecules, RNA‑based drugs and other direct molecular approaches have met with limited success. Vaccination approaches meanwhile have fared much better, entering multiple human studies – albeit thus far they have demonstrated only modest benefits. Despite this, the combination of new generation antitelomerase vaccines with check-point inhibitors is starting to reignite interest in telomerase as a target.
Human telomerase is a ribonucleoprotein complex consisting of two subunits, human telomerase reverse transcriptase (hTERT) and a template component – human telomerase RNA gene (hTERC). The active complex maintains telomerase length via the addition of single stranded TTAGGG sequences to dividing chromosomes, allowing growing cells to avoid senescence pathways triggered by the telomere erosion that occurs as a result of the asymmetrical action of DNA polymerase. Without telomerase activity, each cycle of cell division reduces telomere length by 50‑100 base pairs, effectively placing a limit on cellular mortality. Expressing telomerase, therefore, provides cancer cells with an escape to immortality.
Expression of telomerase
The intriguing notion of telomerase as a universal cancer target stems from the differential expression pattern of the enzyme. hTERT activity is silenced in most normal adult somatic cells, apart from some stem-like cells and proliferating T cells.2 However, it is highly expressed in embryonic tissues and ubiquitous within cancer cells;3 elevated telomerase activity characterises over 90 percent of all cancer types across all stages of tumourigenesis from incipient cancer stem cells to advanced metastatic cells.4 Furthermore, in a variety of tumour types, high telomerase activity correlates with poor patient outcomes; telomerase activity was identified as one of the most important prognostic factors in non-small cell lung cancer.5 For instance, advanced melanoma6 expression of hTERT protein was associated with reduced patient survival.
Another advantage of telomerase as a potential target is the limited potential for tumour resistance mechanisms, as mutations in hTERT leading to loss of function would reduce the proliferative and metastatic potential of the tumour. However, one alternative pathway of telomere elongation has been described, known as alternative lengthening of telomeres (ALT), that has been documented in five to 10 percent of all cancers.7 ALT is uncommon in epithelial malignancies but prevalent in cancers of mesenchymal origin, such as sarcomas, perhaps excluding them from treatments with telomerase-targeted therapies. In all tumour types, however, it appears that switching between telomerase and ALT as a means of maintaining telomere length in tumour cells is a rare phenomenon.
Not only is telomerase expression almost ubiquitous in tumours, it also facilitates an essential function of cancer cells, perhaps their defining feature – the ability to reproduce without limit.
Challenges for direct telomerase inhibitors
Despite telomerase displaying many characteristics of an ideal cancer target and a long history of development, it is not suited in all aspects to conventional drug development processes.
The relatively low abundance of the enzyme has made it difficult to purify and crystallise. Consequently, researchers have been unable to reference the high‑resolution structural models necessary to refine the design of small molecule inhibitors.8
In addition, translational research is hampered by differences in the nature of telomeres and in telomerase expression between humans and standard animal models; the telomeres of inbred mouse strains are five to 10 times longer than those in humans;9 telomerase is widely expressed in the tissues of adult mice10 and not in adult humans. Consequently, standard laboratory animals provide little guidance to the behaviour of potential telomerase-targeted therapeutics in the clinic. Xenograft models that more closely match human tumour complexity might prove more useful.8
One final factor that may have thwarted clinical development is a general misalignment of the therapeutic window for directly acting telomerase inhibitors. Despite cancer cell telomeres being shorter on average than those in matched normal tissues,11 stem cells and T cells may be exposed to toxic inhibitors before any cytocidal impact on the tumour is apparent. Tumour proliferation requires only a low level of telomerase activity.12
Challenges for TERT vaccine development
Immunologists followed a parallel development approach, with the publication of the sequence of hTERT13 adding another target to efforts that had previously been focused on developing vaccines against common driver mutations such as KRAS.14


By permission of Donny Bliss, NIH
Work in vitro had shown that hTERT protein is immunogenic for peripheral blood T lymphocytes from both healthy individuals and cancer patients, suggesting that telomerase is not seen as a self-antigen.
Epitopes within the active site of the hTERT reverse transcriptase were identified by testing the reactivity to candidate epitopes (synthetic long peptides) of T cells from cancer patients. This led to the first therapeutic vaccine targeting telomerase (GV1001), which reached the clinic in 2000 in non‑small cell lung cancer.15
Since that pioneering effort, several different vaccination platforms have been used to develop telomerase vaccines, including peptide vaccines,16,17 autologous dendritic cell vaccines,18 RNA vaccines19 and DNA vaccines.20 Over 30 clinical trials of telomerase vaccines have been completed or are ongoing, with peptide vaccines accounting for approximately two-thirds of them.
However, no telomerase-targeted vaccine has yet been approved. The most advanced candidate is the original GV1001 peptide vaccine, which has a conditional approval in one country, Korea, in a narrow sub‑indication of advanced or metastatic pancreatic cancer.
Nevertheless, within that barren approval landscape there are several important lessons for vaccine design and deployment. Telomerase cancer vaccines appear to be generally safe, with side effects limited to injection site reactions and flu-like symptoms.17 Also, there appears to be considerable scope for vaccine improvement, particularly in peptide vaccines.
At a basic immunological level, the use of longer synthetic peptides opens the possibility of a more rounded immune response through antigen presentation within both HLA class I and class II molecules and therefore both CD8+ (cytotoxic) and CD4+ (helper) T-cell functions.
The precise peptide sequence used can also influence effectiveness. Early clinical trials of GV1001 in several indications, including pancreatic cancer, malignant melanoma and non-small cell lung cancer, showed that patients’ hTERT immune responses extended beyond the direct response to GV1001 to other peptides from the active site region of hTERT, a phenomenon known as intramolecular epitope spreading. The researchers involved21 compared the response in patients who responded well clinically to those who had an immune response to GV1001 but without any evident clinical benefit.21 On that basis, they designed a second-generation hTERT vaccine – UV1 – by selecting three new peptide epitopes from hTERT that were the most frequently recognised.
Another potential avenue for further improvement is the deployment of hTERT vaccines in combination with checkpoint inhibitors. Thus far, while hTERT vaccines have induced high immunological response rates, those effects have been insufficient to control cancer progression. It seems plausible that vaccination-related T-cell expansion has been limited by the activity of CTLA-4 and PD-1/PDL-1 checkpoints. The potential synergy between hTERT vaccines and antibody inhibitors of those checkpoints is being explored in at least nine clinical Phase I/II or Phase II trials, with seven involving the peptide vaccines UV1 and UCPVax and two involving the DNA vaccine INO-5401.
In the next few months, as these studies begin to mature, it is possible that a new landscape of cancer therapies may emerge with telomerase – a near-universal cancer antigen – at its core.
About the author
Dr Jens Bjørheim is the Chief Medical Officer of Ultimovacs, a Norwegian immunotherapy company developing immune stimulatory vaccines for cancer. Jens is a Doctor of Clinical Oncology with an academic research history focused on immunology and cancer genetics. He has extensive experience in the pharmaceutical industry that he gained before joining Ultimovacs.
References
- Kim NW, et al. Specific association of human telomerase activity with immortal cells and cancer. Science. 1994;266:2011–5.
- Morrison SJ, Prowse KR, Ho P, Weissman IL. Telomerase activity in hematopoietic cells is associated with self-renewal potential. Immunity. 1996;5:207–16.
- Hiyama E, Hiyama K. Telomere and telomerase in stem cells. Br J Cancer (2007) 96(7):1020-4.
- Shay JW, Bacchetti S. A Survey of Telomerase in Human Cancer. European Journal of Cancer (1997) 33(5):787-91.
- Taga S, et al. Prognostic impact of telomerase activity in non-small cell lung cancers. Ann Surg (1999) 230(5):715-20.
- Hugdahl E, et al. Prognostic impact and concordance of TERT promoter mutation and protein expression in matched primary and metastatic cutaneous melanoma. Br J Cancer (2018) 118(1):98-105.
- Heaphy CM, et al. Prevalence of the alternative lengthening of telomeres telomere maintenance mechanism in human cancer subtypes. Am J Pathol (2011) 179(4):1608-15.
- Guterres AN, Villanueva J. Targeting telomerase for cancer therapy. Oncogene (2020) 39:5811–5824.
- Hemann MT, Greider CW. Wild-derived inbred mouse strains have short telomeres. Nucleic Acids Res. 2000;28:4474–8.
- Greenberg RA, et al. Expression of mouse telomerase reverse transcriptase during development, differentiation and proliferation. Oncogene. 1998;16:1723–30.
- Barthel FP, et al. Systematic analysis of telomere length and somatic alterations in 31 cancer types. Nat Genet. 2017;49:349–57.
- Ouellette MM, et al. Subsenescent telomere lengths in fibroblasts immortalized by limiting amounts of telomerase. J Biol Chem. 2000;275:10072–6.
- Harrington L, et al. Human telomerase contains evolutionarily conserved catalytic and structural subunits. Genes Dev. 1997;11(23):3109–15.
- Gjetrsen MK, et al. Vaccination with mutant ras peptides and induction of T-cell responsiveness in pancreatic carcinoma patients carrying the corresponding RAS mutation, The Lancet 1995; 346: 1399-1400
- Brunsvig PF, et al. Telomerase peptide vaccination: a phase I/II study in patients with non-small cell lung cancer. Cancer Immunol Immunother 55, 1553–1564 (2006).
- Rosalia RA, et al. Dendritic cells process synthetic long peptides better than whole protein, improving antigen presentation and T-cell activation. European Journal of Immunology (2013) 43(10):2554-65.
- Negrini S, De Palma R, Filaci G. Anti-cancer Immunotherapies Targeting Telomerase. Cancers (2020) 12(8):2260.
- Mantia-Smaldone GM, Chu CS. A Review of Dendritic Cell Therapy for Cancer: Progress and Challenges. BioDrugs (2013) 27(5):453-68.
- Rittig SM, et al. Intradermal Vaccinations with RNA Coding for TAA Generate CD8+ and CD4+ Immune Responses and Induce Clinical Benefit in Vaccinated Patients. Molecular Therapy (2011) 19(5):990-9.
- Teixeira L, et al. A First-in-Human Phase I Study of INVAC-1, an Optimized Human Telomerase DNA Vaccine in Patients with Advanced Solid Tumours. Clinical Cancer Research (2020) 26(3):588-97. doi: 10.1158/1078-0432.Ccr-19-1614.
- Inderberg-Suso E-M, et al. Widespread CD4+ T-cell reactivity to novel hTERT epitopes following vaccination of cancer patients with a single hTERT peptide GV1001. Oncoimmunology vol. 1,5 (2012): 670-686.
Related topics
Cancer Research, Research & Development, Targets, Vaccine
Related conditions
Cancer Research, metastatic pancreatic cancer, Non-small cell lung cancer (NSCLC)
Related organisations
Sartorius