The potential of epigenomic medicine in cardiology
Posted: 22 June 2021 | Professor John Giannios (Learna) | No comments yet
Advances in genomic medicine are playing an increasingly important role in the field of cardiology. Better analysis and understanding of patient genomic and epigenomic information can enable more personalised patient treatment and medical intervention. Here, Professor John Giannios considers the potential to use genomic medicine to prevent, monitor, diagnose and treat cardiovascular diseases.
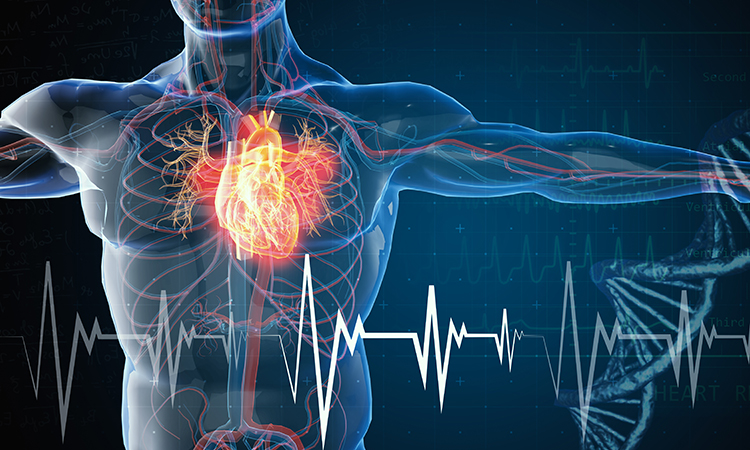
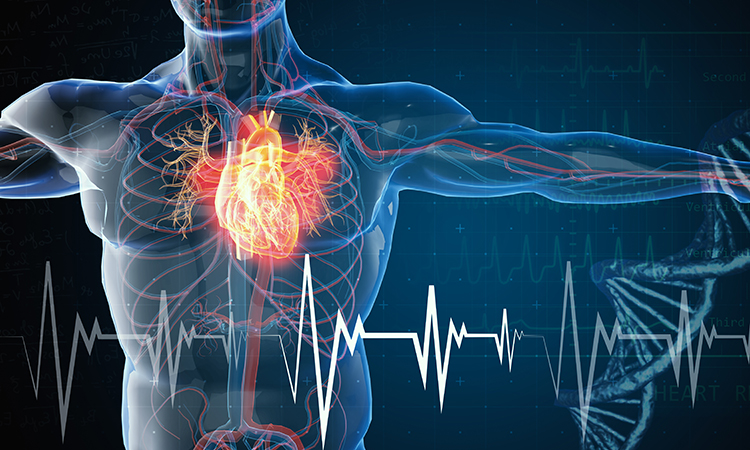
Cardiovascular diseases (CVDs) remain the number one cause of global death, killing approximately 18 million patients annually. Unfortunately, these numbers are increasing constantly along with disability adjusted life years (DALYs) – particularly in developed countries. We urgently need new screening, diagnostic, preventive, monitoring and mainly therapeutic approaches based on genomic medicine against CVDs.
CVDs are multifactorial-disorders and beyond their genetic/genomic pathogenic information which is encoded in the DNA sequence, is the epigenetic/epigenomic pathogenic etiology consisting of protein, RNA and DNA modifications which may be targeted for personalised approaches in the screening, diagnosis, prevention, monitoring and treatment of CVDs. Consequently, we can implement personalised, evidence-based medicine by associating the clinical phenotype of each CVD patient to their disease-related genotype and epigenotype.
In an irreversible process, disease‑related deleterious variants in the DNA, with errors in the order of nucleotides, may lead to faulty coding of instructions, leading to proteins with deregulated functions. Moreover, there are additional pathogenic factors combined with the alterations in DNA sequences involving the genetic variation of inherited alterations in mitosis and meiosis, which influence disease-related gene expression and function. These factors are epigenetic and epigenomic which may transmit pathogenic information by macromolecules when DNA is copied into RNA via transcription, which synthesises proteins in the translation process. The deregulated epigenetic components leading to the pathogenesis of CVDs consist of DNA modifications that can be caused by deleterious environmental and lifestyle factors. This can lead to covalent DNA methylation of cytosines, mainly in CpG‑dinucleotides that inactivate transcription and deregulate gene expression that can interact with multiomic pathways, including transcriptomics, metabolomics, nutrigenomics, lipidomics, epigenomics, microbiomics, immunogenomics, inflammomics, epitranscriptomics, genomics and other omics.
…novel treatments based on epigenetics and epigenomics may target the abnormal expression of disease‑ causing protein-coding genes in CVDs”
This pathogenic multifactorial process contributes to the development of CVDs, including cardiac hypertrophy, hypertension, coronary heart disease, myocardial infarction, immune‑related inflammation contributing to atherosclerosis, heart failure and cardiac remodelling. This can lead to ventricular dysfunction and malignant arrhythmias that can be reversed to improve cardiac function. Methylation of chromatin remodelling is regulated by enzymes such as DNA methyltransferase (DNMT), which adds a methyl-group to position-5 of the cytosine-ring in the CpG-dinucleotide after the administration of demethylating agents, such as azanuclosides. This can cause hypomethylation, allowing the binding of transcription factors and transcription of epigenetically-silenced genes. Thus, DNMT inhibitors may reverse methylation of cytosine-residues reactivating transcription and switching genes on.
The other epigenetic player involves RNA, including the RNA-dependent DNA methylation (RdDM) which mediates the specific targeting of the DNMT enzymes to genomic sequences. After the activation of RdDM by the transcription process of the genomic locus, the synthesised RNA – with the action of RNA-dependent RNA polymerases – is converted into double stranded RNA (dsRNA). This activates RNA interference for precise post-transcriptional gene silencing (PTGS) with small inhibitory RNA (siRNA) or interference RNA which disrupts the process of protein synthesis by degradation of mRNA. Non-coding RNA, such as microRNA (miRNA) or antisense RNA, share the same RNA‑induced silencing-complex (RISC) as siRNA, but they use translational‑repression for silencing gene expression after binding to the targeted mRNA’s 3’ untranslated region (UTR) that leads to the inhibition of protein translation.
Another significant epigenetic component consists of RNA editing that may be involved in the pathogenesis of CVD by direct alteration of RNA nucleotides with no changes in the DNA sequence. It could lead to changes of protein coding transcripts including non‑coding RNA. The resulting RNA mutations may destruct or generate splice sites and influence codon usage, leading to the synthesis of novel disease‑causing proteins. Furthermore, RNA variants can lead to the alteration of RNA stability and regulation involving target diversity and action of non-coding RNA. Aside from this, enzymes including apolipoprotein-B mRNA‑editing enzyme catalytic‑polypeptide (APOBEC) can cause mutations in the cytosine residues to uracil (or C to U) with the most common form consisting of adenosine-deaminases acting on RNA (ADARS) potentially leading to mutations in adenosine residues in RNA to inosine (or A to I). RNA modifications, including RNA editing, may be detected by RNA sequencing. RNA editing has been linked to patients with the clinical phenotype of atherosclerotic vascular diseases including advanced carotid atherosclerotic disease (ACAD), coronary artery disease (CAD), aortic aneurysms and atherosclerosis.1
Another important epigenomic component consists of protein epigenetics. A proteome can be modified by alterations in the DNA sequence and it can accumulate epigenetic information leading to a disease-related clinical phenotype without altering the DNA sequence in any way. Post-translational modifications (PTMs) of histones, including methylation and acetylation with the action of enzymes consisting of erasers and writers, may recruit regulatory molecules and change the structure of chromatin, leading to regulation of gene expression in a reversible and plastic way. Histone deacetylase inhibitors (HDACi), histone acetyltransferases inhibitors (HATi), histone methyltransferase inhibitors (HMTi) and bromodomain inhibitors have the therapeutic potentiality to prevent or treat cardiovascular diseases and improve cardiac-functions. Some examples include:
- Apicidin – an HDACi that has exhibited reduction of myocardial hypertrophy
- Givinostat – a pan-HDACi that reduces inflammatory response, EMT and cardiac-fibrosis
- Vorinostat – an HDACi that decreases infarct-size, improves cardiac function, reduces cardiac hypertrophy and improves survival-rate
- SK7041 – an HDACi that decreases myocardial hypertrophy
- JQ1 – a chromatin acetylation bromodomain inhibitor that improves cardiac function and suppresses pathological cardiac remodelling and cardiac hypertrophy
- Curcumin – an HATi for p-300 that prevents the deterioration of systolic function and heart failure.2
Another form of protein epigenetics involves a complex of protein groups including Trithorax (TrxG) proteins which methylate lysine-4 on H3, leading to the activation of gene expression and Polycomb (PcG) proteins which methylate lysine-27 on H3, leading to the repression of gene expression. Both protein complexes consist of histone erasers, writers or chromatin‑remodelling proteins including complexes NURF, ISWI and SWI-SNF. These protein complexes act via DNA elements termed Polycomb‑Trithorax Response-Elements (PRE). TrxG proteins may be misregulated or mutated in many diseases and most likely in CVDs. Thus, potential therapeutic approaches could include small molecule inhibitors against SWI-SNF mutated-targets. Both complexes of TrxG and PcG are necessary for heart development, proliferation and regulation of adult stem cells. These are vital for improving longevity and the anti-ageing phenotype. BMI-1, a member of the PcG-complex, may also protect against hypertrophy and dilated cardiomyopathy or DCM.3
Another significant epigenetic component consists of RNA editing that may be involved in the pathogenesis of CVD by direct alteration of RNA nucleotides”
Furthermore, significant epigenetic mechanisms involved in the pathogenesis of CVDs include mitochondrial DNA methylation and adenosine triphosphate (ATP) dependent chromatin conformation and remodelling complexes.
Epigenomic medicine approaches have the potential to regulate the expression of disease-causing protein-coding genes, leading to personalised evidence‑based approaches involving screening, prevention, monitoring, diagnosis and mainly treatment against cardiovascular diseases. Delivery and targeting of epidrugs by nanosomal systems may reduce systemic toxicity and enhance therapeutic index. The implementation of next-generation sequencing (NGS) for the analysis of epigenetic components including non-coding RNA genes may lead to the discovery of novel disease‑related deleterious variants associated to clinical phenotypes. After validation, they may be used for personalised medicine approaches mediated by targeted epidrugs that could be tailored to each patient or group of patients with the same epigenomic characteristics as a stratified medicine approach in cardiology. We already know numerous epigenomic components that regulate the disease‑causing expression of protein‑coding genes involved in the pathogenesis of cardiovascular diseases, which may be targeted with epidrugs. Generally, these approaches against CVDs might not be used against other diseases such as cancer where therapeutic approaches aim to induce apoptosis or type I programmed cell death (PCD) and inhibit angiogenesis. These do not apply to cardiology because in phases of acute or subacute myocardial‑infarction, we must inhibit apoptosis in cardiomyocytes which has been induced by hypoxia and for reducing the damage that has been caused by the myocardial infarction, we must induce angiogenesis for counteracting hypoxia and its subsequent damaging effects.
Thus, novel treatments based on epigenetics and epigenomics may target the abnormal expression of disease‑causing protein-coding genes in CVDs, especially with the reversal of epigenetic alterations and manipulation of the disease-related expression of non‑coding RNA genes.
About the author
Professor John Giannios is a specialist in genomic medicine and is the Programme Leader in Postgraduate Genomic Medicine & Healthcare at online learning provider, Learna. He is also a member of the British Society of Genetic Medicine and the Clinical Genetics Society as well as a Fellow of the Genomic Medicine Foundation. He regularly writes and reviews articles relating to genomic medicine for medical journals in his role as Editor of the Open Access Journal of Surgery.
References
- Uchida S, Jones PJ. RNA editing: Unexplored opportunities in the cardiovascular system. Circ Res. 2018 February 02; 122 (3): 399-401.
- Liu FC, Tang HWW. Epigenetics in Cardiac Hypertrophy and Heart Failure. JACC: Basic to Translational Science. 2019; 4(8): 976-993.
- Kim SY, Morales CR, Gillette TG, Hill JA. Epigenetic regulation in heart failure. Curr Opin Cardiol. 2016 May;31(3):255-65. doi: 10.1097/ HCO.0000000000000276. PMID: 27022893; PMCID: PMC4955576.
Related topics
Drug Development, Epigenetics, Genetic analysis, Genomics, Molecular Targets, Personalised Medicine
Related conditions
Advanced carotid atherosclerotic disease (ACAD), Atherosclerosis, Cancer, cardiac hypertrophy, cardiovascular disease (CVD), Coronary artery disease (CAD), Coronary heart disease, fibrosis, Hypertension, myocardial infarction
Related organisations
GenScript