Oncology biomarkers in clinical practice – a new horizon
Posted: 4 May 2014 | Heidi Olzscha (University of Oxford), Nicholas B. La Thangue (University of Oxford), Semira Sheikh (University of Oxford)
The ability to individualise a patient’s care has become a particularly pressing issue in the field of cancer therapeutics as there is a growing recognition that both the global incidence of cancer is steadily rising and the associated costs in terms of treatment and lost productivity are ever increasing. Advances in molecular profiling, which allow the typing of cancers according to their gene expression profile, have made it possible to identify biomarkers that have the potential to allow risk stratification of patients into disease-and treatmentspecific subgroup…
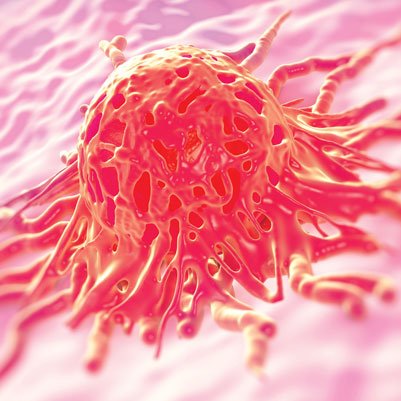
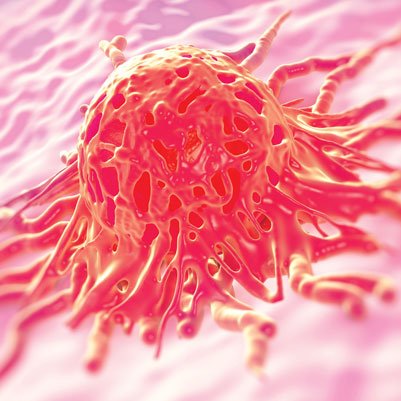
In an era where medicine is increasingly underpinned by a molecular understanding of disease and an evolving evidence base for best practice, the concept of personalised medicine, where treatment selection for each patient becomes individualised, has gained momentum1. The ability to individualise a patient’s care has become a particularly pressing issue in the field of cancer therapeutics as there is a growing recognition that both the global incidence of cancer is steadily rising and the associated costs in terms of treatment and lost productivity are ever increasing2. Advances in molecular profiling, which allow the typing of cancers according to their gene expression profile, have made it possible to identify biomarkers that have the potential to allow risk stratification of patients into disease-and treatment-specific subgroups1,2.
Cancer biomarkers have been generally classified into three different types: prognostic, pharmacodynamic and predictive. Prognostic biomarkers can give information on the natural course of a given disease and may be useful in guiding the extent of therapy required. Pharmacodynamic biomarkers can assess the impact of a drug on a disease by measuring, for instance, the level of proliferation and/or cell death of a tumour in response to a drug. By contrast, predictive biomarkers allow a prospective assessment of whether a particular cancer may or may not respond to a given drug, thereby giving the opportunity of tailoring treatment and administering highly targeted therapies where in the past systemic chemotherapeutic agents would have been used.
Biomarkers in solid tumours
The concept of using biomarkers for the stratification of patients in oncology was pioneered in solid tumours, and today breast cancer patients are routinely stratified according to their HER2 and ER status4. Over 75 per cent of breast cancers have been shown to express oestrogen receptor alpha (ERα). Several randomised controlled trials introducing the selective oestrogen receptor modulator tamoxifen as adjuvant hormonal therapy in early and advanced breast cancer subsequently demonstrated prolonged progression-free and overall survival in those patients with ERα-positive disease compared with those who were ERα-negative5,6.
Although tamoxifen has become the mainstay of hormone treatment for breast cancer over the last 20 years, it is increasingly apparent that there is considerable heterogeneity in response. Thus, ERα positivity is not synonymous with sensitivity to treatment, and even patients who do respond well initially may relapse later on whilst still expressing functional ERα. Consequently, there remains a need for biomarkers that can help to improve the selection of patients likely to respond to hormone treatment1,5.
The epidermal growth factor receptor (EGFR) is a cell surface receptor which belongs to the HER/ERB family of receptor kinases and promotes cell proliferation and survival through the MAPK, PI3K and JAK/STAT pathways1,7. It is frequently mutated in different cancers but has been most successfully targeted in colorectal cancer. Two monoclonal antibodies with anti-EGFR activity, cetuximab and panitumumab, have been approved for the treatment of metastatic, chemo-refractory colorectal cancer, leading to responses in approximately 10 per cent of patients1. Interestingly, early studies did not reveal a linear relationship between EGFR expression and response to EGFR inhibitors, and a systematic review that retrospectively analysed patient subsets from Phase II and III trials found that patients with co-existing KRAS mutations did not receive any benefit from treatment with cetuximab or panitumumab1,6,8. This finding has established the KRAS mutation status as an important biomarker for predicting disease response to EGFR inhibitor therapy in colorectal cancer8. More recently, other mutations in KRAS and mutations affecting genes involved in downstream signalling from KRAS, such as BRAF, have been implicated in resistance to EGFR therapy, thus highlighting the need for a comprehensive risk-stratification and patient selection approach in these cancer subgroups6.
NSCLC (non-small cell lung cancer) is another type of solid tumour for which biomarkers are applied in the clinic, and especially mutations in KRAS play an important role and can be assessed nowadays9. NSCLC can be treated with chemotherapeutics such as cisplatin or etoposide. For patients with advanced lung cancers who meet certain criteria, a targeted therapy drug such as bevacizumab or cetuximab may be added to treatment as well. However, the tyrosine kinase inhibitor gefitinib is more effective, if the corresponding patients have mutations in the EGFR gene10. By comparison, the development of other biomarkers in solid tumours has markedly lagged behind. The diagnostic marker CA-125 is considered in Phase III trial inclusion criteria to confirm diagnosis of ovarian cancer11, whereas the most often applied biomarker in prostate cancer is the prostate specific antigen (PSA), which has been routinely used in screening and diagnosis of prostate cancer, although false-positive diagnoses make the development of alternative biomarkers desirable12. Examples of clinically applicable biomarkers in melanomas are for example BRAF13 and c-KIT14, whereas c-KIT activation is also a ubiquitous feature of gastrointestinal stromal tumours15. Clinically useful biomarkers for pancreatic cancer are rare, but include Ep-Cam or Ep-Cam positive cells16, and CA19-917.
In summary, the application of biomarkers to various solid tumour types may allow better classification of heterogeneous disease and therefore a more targeted treatment approach.
Biomarkers in haematological malignancy
Advances in discovering the cytogenetic and molecular lesions responsible for the pathogenesis of haematological malignancies have made it possible to provide both prognostic information and to drive forward the development of risk-stratified approaches in treatment.
Acute myeloid leukaemia (AML) is a case in point. AML comprises a highly heterogeneous group of malignant clonal disorders which arise from haematopoietic progenitors, ultimately leading to the outgrowth of myeloid blasts and bone marrow failure. Approximately 60 per cent of AML patients have been found to exhibit an abnormal karyotype. In up to 10 per cent of AML cases in children and younger adults rearrangements of the retinoic acid receptor (RARA) gene on chromosome 17q21 lead to a phenotype of acute promyelocytic leukaemia (APML). The vast majority of APML cases harbour the translocation t(15;17) involving the PML gene on chromosome 157.
The PML-RARA fusion protein is involved in transcriptional repression of target genes which results in differentiation arrest and proliferation of promyelocytes7,18. This can be overcome by the administration of all-trans-retinoic acid (ATRA). In pharmacological doses, ATRA induces a conformational change in the PML-RARA fusion protein, causing the release of co-repressors and the recruitment of coactivators. This switch allows active transcription of genes repressed by PML-RARA, resulting in rapid terminal cell differentiation and disease remission. However, in clinical practice, ATRA by itself rarely leads to prolonged remission, and the molecular basis for this phenomenon has not been completely resolved. The standard of treatment, therefore, has traditionally been a combination of ATRA with an anthracycline-based chemotherapy regimen, with cure rates exceeding 80 per cent18. More recently, trials of ATRA with arsenic trioxide have been shown to have similar efficacy and reduced haematologic toxicity in patients with low-to-intermediate risk APML, suggesting that this disease in the future may become curable without conventional chemotherapy19. The ability to detect the t(15;17) translocation event in a subset of AML patients has thus provided a widely used predictive biomarker for diagnosis and treatment.
In those AML patients with a normal karyotype (approximately 40 per cent), the mutation status of certain genes such as Fms-like tyrosine kinase 3 (FLT3), has been shown to be of major importance with regards to pathogenic and prognostic outcome7,20. FLT3 is mutated in approximately one third of AML cases. The majority of mutations are internal tandem duplications (ITDs) that lead to constitutive activation of the receptor and are often associated with higher presenting white cell counts. The presence of FLT-ITD has been shown to provide a major independent adverse prognostic indicator, related to the propensity for early disease relapse and poorer overall survival20.
There is thus a strong rationale for developing tyrosine kinase inhibitors (TKIs) that target FLT3. In recent years, FLT3 inhibitors such as quizartinib and sorafenib have shown promising results in clinical trials, with some reports of bone marrow remission. For instance, investigators examining the effects of crenalonib, a presumed type I TKI which can bind to both the active and inactive conformations of the FLT3 tyrosine kinase receptor, were able to demonstrate that dual therapy with type I and type II TKIs may have greater anti-leukaemic activity and greater clinical effectiveness21,22.
Detecting the FLT-ITD mutation in a newly diagnosed AML patient therefore allows early risk-stratification and the timely introduction of molecular targeted therapy alongside a conventional chemotherapy regimen.
Evolving biomarkers
It has become evident over the past two decades that many biological processes are involved in tumour initiation and progression. Besides underlying mutations in crucial oncogenes or tumour suppressor genes, processes such as the cell cycle, DNA repair, angiogenesis or autophagy can influence the progression of cancer and therefore affect apoptosis23. These processes are not only determined by the expression level of certain proteins, but also by post-translational protein modifications which include phosphorylation, ubiquitination or acetylation24. Acetylation and deacetylation of proteins at the ε-amino group of lysine residues are executed by histone acetyltransferases (HATs) and their functional antagonists histone deacetylases (HDACs), respectively25. Aberrant acetylation in many tumour cell types, and the fact that acetyl residues can influence chromatin packaging and density and therefore gene expression, led to the use of HDAC inhibitors (HDIs) to treat certain malignancies26. HDIs induce apoptosis, and two in particular, vorinostat (SAHA, Zolinza) and romidepsin (FK228, Istodax) have already been approved for the treatment of cutaneous T-cell lymphoma (CTCL)27.
In order to identify a biomarker to predict for sensitivity to HDI therapy, a shRNA screen was carried out. One of the proteins found in this screen was HR23B (human UV excision repair protein RAD23 homolog B), which is involved in DNA repair and the shuttling of proteins to the proteasome28. The predictive power of HR23B for clinical response to HDAC inhibitors was investigated through the analysis of a unique collection of CTCL biopsies taken from a Phase II clinical trial, where a coincidence between high HR23B expression and clinical response to HDAC inhibitors was found29. Recently, it has also been shown that downregulation of the proteasomal shuttling factor HR23B occurs via the BUZ domain of histone deacetylase HDAC6, which leads to a reduction of ubiquitination levels of proteins. HSP90 was identified as a key effector of HDAC6 on HR23B levels, embedded in a network of proteins involved in protein quality control30.
Besides HR23B, which turned out to be useful as a predictive and responsive biomarker, HDACs themselves may be used to monitor HDI activity or predict a potential clinical outcome. Examples are increased levels of HDAC1 in gastric cancer and hormone-refractory prostate cancer31,32, and higher expression of HDAC2 and HDAC3 in colon cancer33,34. Other markers for the post-translational event of acetylation and hyperacetylation upon HDI treatment could be histones, where acetylation can be detected via immunoblotting, immunohistochemistry or mass spectrometry35. Similar studies are carried out to determine the role of other posttranslational modifications in tumour progression, and to identify potential biomarkers36.
Outlook
One of the upcoming challenges will be to identify and treat different types of cancer in different ways and ideally to personalise cancer treatment (Figure 1)2. The analysis of different biomarker patterns in order to obtain a molecular signature of an individual person or an integrative personal omics profile (iPOP) could have a major impact on therapeutic strategies. However, it is envisaged that the more complex the biomarker pattern will be, the more complex the respective therapeutic options will become. So called dual targeting agents, some of them under development, could facilitate managing this complexity. An example of a dual targeting agent is a bivalent compound which inhibits histone deacetylases and topoisomerase II37.
‘Theranostics’, a portmanteau of therapeutics and diagnostics, describes an important aspect of biomarker research and applied therapeutics, with the aim of streamlining treatment for each individual patient and minimising side effects by aligning the right patient with the right drug. Although there remains a lot of research to be done in biomarker discovery, successful examples in oncology have shown that their use has contributed to diagnostic and treatment strategies which ultimately increase life expectancy and quality of life for patients. The combined effort of researchers and clinicians in developing new biomarkers and individual risk profiles will hopefully transform the practice of medicine.
Acknowledgement
The research was supported by MRC and CRUK (programme grant C300/A13058). Heidi Olzscha was supported by an EMBO Long-Term Fellowship; Semira Sheikh was supported by an Anya Sturdy Fellowship.
References
- La Thangue, N.B. and D.J. Kerr, Predictive biomarkers: a paradigm shift towards personalized cancer medicine. Nat Rev Clin Oncol, 2011. 8(10): p. 587-96
- Olzscha, H., M. New, and N.B. La Thangue, Personalised Cancer Medicine: Fulfilling the Promise, in Encylopedia of Life Sciences. 2013, John Wiley & Sons Ltd, Chichester
- Kalia, M., Personalized oncology: recent advances and future challenges. Metabolism, 2013. 62 Suppl 1: p. S11-4
- Nielsen, T.O., et al., Immunohistochemical and clinical characterization of the basal-like subtype of invasive breast carcinoma. Clin Cancer Res, 2004. 10(16): p. 5367-74
- Patani, N. and L.A. Martin, Understanding response and resistance to oestrogen deprivation in ER-positive breast cancer. Mol Cell Endocrinol, 2014. 382(1): p. 683-94
- Duffy, M.J., N. O’Donovan, and J. Crown, Use of molecular markers for predicting therapy response in cancer patients. Cancer Treat Rev, 2011. 37(2): p. 151-9
- Arber, D., R. Brunning, and M. Le Beau, Acute myeloid leukaemia with recurrent genetic abnormalities, in WHO classification of Tumours of Haematopoietic and Lymphoid Tissues, W. OMS, Editor. 2008
- Allegra, C.J., et al., American Society of Clinical Oncology provisional clinical opinion: testing for KRAS gene mutations in patients with metastatic colorectal carcinoma to predict response to anti-epidermal growth factor receptor monoclonal antibody therapy. J Clin Oncol, 2009. 27(12): p. 2091-6
- Linardou, H., et al., Assessment of somatic k-RAS mutations as a mechanism associated with resistance to EGFR-targeted agents: a systematic review and meta-analysis of studies in advanced non-small-cell lung cancer and metastatic colorectal cancer. Lancet Oncol, 2008. 9(10): p. 962-72
- Azzoli, C.G., et al., American Society of Clinical Oncology Clinical Practice Guideline update on chemotherapy for stage IV non-small-cell lung cancer. J Clin Oncol, 2009. 27(36): p. 6251-66
- Zhang, Z., et al., Three biomarkers identified from serum proteomic analysis for the detection of early stage ovarian cancer. Cancer Res, 2004. 64(16): p. 5882-90
- Stamey, T.A., et al., Prostate-specific antigen as a serum marker for adenocarcinoma of the prostate. N Engl J Med, 1987. 317(15): p. 909-16
- Davies, H., et al., Mutations of the BRAF gene in human cancer. Nature, 2002. 417(6892): p. 949-54
- Curtin, J.A., et al., Somatic activation of KIT in distinct subtypes of melanoma. J Clin Oncol, 2006. 24(26): p. 4340-6
- Rubin, B.P., et al., KIT activation is a ubiquitous feature of gastrointestinal stromal tumors. Cancer Res, 2001. 61(22): p. 8118-21.
- Fong, D., et al., Ep-CAM expression in pancreatic and ampullary carcinomas: frequency and prognostic relevance. J Clin Pathol, 2008. 61(1): p. 31-5
- Koopmann, J., et al., Serum diagnosis of pancreatic adenocarcinoma using surface-enhanced laser desorption and ionization mass spectrometry. Clin Cancer Res, 2004. 10(3): p. 860-8
- Ablain, J. and H. de The, Revisiting the differentiation paradigm in acute promyelocytic leukemia. Blood, 2011. 117(22): p. 5795-802
- Lo-Coco, F., et al., Retinoic acid and arsenic trioxide for acute promyelocytic leukemia. N Engl J Med, 2013. 369(2): p. 111-21
- Scholl, C., D.G. Gilliland, and S. Frohling, Deregulation of signaling pathways in acute myeloid leukemia. Semin Oncol, 2008. 35(4): p. 336-45
- Fathi, A.T., Emergence of crenolanib for FLT3-mutant AML. Blood, 2013. 122(22): p. 3547-8
- Zimmerman, E.I., et al., Crenolanib is active against models of drug-resistant FLT3-ITD-positive acute myeloid leukemia. Blood, 2013. 122(22): p. 3607-15
- Hanahan, D. and R.A. Weinberg, The hallmarks of cancer. Cell, 2000. 100(1): p. 57-70
- Krueger, K.E. and S. Srivastava, Posttranslational protein modifications: current implications for cancer detection, prevention, and therapeutics. Mol Cell Proteomics, 2006. 5(10): p. 1799-810
- New, M., H. Olzscha, and N.B. La Thangue, HDAC inhibitor-based therapies: can we interpret the code? Mol Oncol, 2012. 6(6): p. 637-56
- Inche, A.G. and N.B. La Thangue, Chromatin control and cancer-drug discovery: realizing the promise. Drug Discov Today, 2006. 11(3-4): p. 97-109
- Khan, O. and N.B. La Thangue, HDAC inhibitors in cancer biology: emerging mechanisms and clinical applications. Immunol Cell Biol, 2012. 90(1): p. 85-94
- Fotheringham, S., et al., Genome-wide loss-of-function screen reveals an important role for the proteasome in HDAC inhibitor-induced apoptosis. Cancer Cell, 2009. 15(1): p. 57-66
- Khan, O., et al., HR23B is a biomarker for tumor sensitivity to HDAC inhibitor-based therapy. Proc Natl Acad Sci U S A, 2010. 107(14): p. 6532-7
- New, M., et al., A regulatory circuit that involves HR23B and HDAC6 governs the biological response to HDAC inhibitors. Cell Death Differ, 2013. 20(10): p. 1306-16
- Choi, J.H., et al., Expression profile of histone deacetylase 1 in gastric cancer tissues. Jpn J Cancer Res, 2001. 92(12): p. 1300-4
- Halkidou, K., et al., Upregulation and nuclear recruitment of HDAC1 in hormone refractory prostate cancer. Prostate, 2004. 59(2): p. 177-89
- Zhu, P., et al., Induction of HDAC2 expression upon loss of APC in colorectal tumorigenesis. Cancer Cell, 2004. 5(5): p. 455-63
- Wilson, A.J., et al., Histone deacetylase 3 (HDAC3) and other class I HDACs regulate colon cell maturation and p21 expression and are deregulated in human colon cancer. J Biol Chem, 2006. 281(19): p. 13548-58
- Wu, Q., et al., SAHA treatment reveals the link between histone lysine acetylation and proteome in nonsmall cell lung cancer A549 Cells. J Proteome Res, 2013. 12(9): p. 4064-73
- Wulfkuhle, J.D., et al., New approaches to proteomic analysis of breast cancer. Proteomics, 2001. 1(10): p. 1205-15
- Guerrant, W., et al., Dual targeting of histone deacetylase and topoisomerase II with novel bifunctional inhibitors. J Med Chem, 2012. 55(4): p. 1465-77
Related topics
Biomarkers, Oncology, Personalised Medicine
Related conditions
Breast cancer
Related organisations
Oxford University
Related people
Heidi Olzscha, Nicholas B. La Thangue, Semira Sheikh