CRISPR: kick-starting the revolution in drug discovery
Posted: 13 December 2019 | Dr Pushpanathan Muthuirulan (Harvard University) | No comments yet
Gene editing using the CRISPR system has been established as the most powerful tool in the search for new drugs and is now being exploited for therapeutic purposes. Here, Pushpanathan Muthuirulan discusses the promises and wider opportunities of using CRISPR technology to open up the possibility of large-scale screening of drug targets. He also highlights the importance of implementing CRISPR technology into clinical practice for development of next-generation therapeutics and patient-tailored medicine.
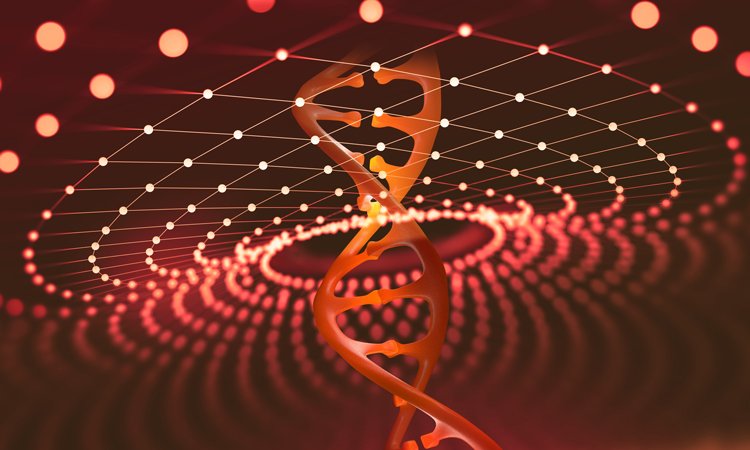
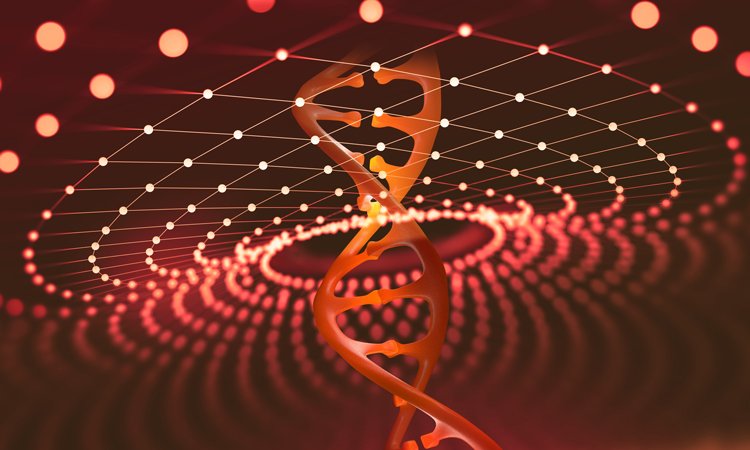
THE DRUG discovery process, in which new drug candidates are discovered and evaluated for therapeutic use, has resulted in both promising and life-saving therapies for numerous diseases including inherited genetic disorders and pathogenic infections.1 However, the discovery and testing of a new drug candidate typically takes more than a decade and the total cost associated with drug discovery processes can exceed $1 billion.2 In the United States, the drug discovery process takes an average of 12 years and in excess of $1 billion to develop a new drug.3 Furthermore, only a few drug candidates actually make it to market; the chance of a new drug actually reaching market is only one in 5,000. The high cost and lengthy effort of getting new drugs to market make the drug development process a risky endeavour for pharmaceutical companies, which consequently hinders discovery and development of new therapies. The recent emergence of genome editing technologies and advances in our understanding of human genome sequences have raised hope that direct manipulation of the genome could potentially revolutionise the process of drug discovery and therapeutics.4 In particular, new technologies like CRISPR-Cas9 are key to unlocking potential drug targets and could have a profound impact on modern drug discovery and development.1,5
CRISPR (clustered regularly interspaced short palindromic repeats) is a genome-editing technology that enables scientists to precisely alter a piece of DNA sequence in the genome of any organism. The CRISPR method was unveiled in 2012 and has now become an indispensable tool in biological research. CRISPR is much cheaper, faster, more accurate and efficient than other genome editing methods. CRISPR technology allows a huge range of alterations to be made in genomes, including gene knockouts, knock-ins, generation of specific insertions or mutations and the ability to activate or repress gene transcription. These changes coupled with physiological relevance make this technology more likely to have a tremendous impact across the entire breadth of the drug discovery process.6 Most scientists have been using CRISPR in cultured cells and animal models to understand a wide variety of diseases, including haemophilia, cystic fibrosis, osteoarthritis and sickle cell disease. This technology offers the promise to cure complex diseases such as human immunodeficiency virus (HIV) infection, cancer, heart disease and mental illnesses.7 Scientists are still working hard to demonstrate the safety and efficacy of this technology for use in humans. However, ethical concerns arise when using CRISPR technology to alter human genomes; specifically, editing genomes in germline cells and embryos brings up several ethical challenges and these practices are currently banned in many countries. Even though CRISPR has some disadvantages that hinder the prospective application of this technology in clinical practice, it has tremendous potential to transform medicine, enabling us to not only treat diseases but also prevent them completely.8
CRISPR-based drug discovery
The drug discovery process requires several steps before new drugs are approved for clinical use. The most common pre-clinical process in drug discovery involves drug target identification and validation, high-throughput screening (HTS), hit validation and optimisation of the lead drug molecule. CRISPR is accelerating all stages of the drug discovery process and advancing the stages of pre-clinical drug development.
i) CRISPR-based drug target identification
High-throughput functional genomics screens using CRISPR-Cas9 provide affordable access to large numbers of drug targets, advancing the search for potential therapeutic candidates”
The first step in drug discovery involves identification of a potential drug target linked to a disease of interest. Putative drug targets are discovered in several ways, often stemming from academic research labs. High-throughput functional genomics screens using CRISPR-Cas9 provide affordable access to large numbers of drug targets, advancing the search for potential therapeutic candidates. It also unravels the potential connection between genotype and biological phenotype giving scientists the ability to scan whole genomes to investigate the functional consequences of gene expression. CRISPR has been more commonly used to systematically knock out large numbers of candidate genes in the laboratory, offering promise for accelerating the development of new therapeutic drugs.9 It also helps in unravelling new drug targets to establish a strong causative relationship between targets and diseases.
ii) CRISPR-based compound screening
The next step in the drug discovery process following target identification and validation is to examine millions of compounds using HTS. The high-throughput compound screening methods include biochemical screens and cell‑based screens. Biochemical screens mainly involve assessment of interaction and binding affinities between compounds and targets, whereas cell-based screens leverage knowledge of functional relationships of such interactions. In recent years, CRISPR has improved cell-based screening and offers tremendous opportunities for researchers to generate mutant cell lines that mimic disease phenotypes. Moreover, libraries of guide RNA (sgRNA) targeting various genomic loci in a single pool of cells can facilitate the generation of thousands of genetic perturbations in a cell population that can be evaluated further for specific phenotypes implicating cellular processes and gene functions in an unbiased systemic way.10 Thus, generation of better cellular models using CRISPR technology would allow researchers to perform HTS of compounds resulting in identification of the most effective drug molecule for therapeutics. This framework could revolutionise drug design, save time, reduce cost and accelerate the drug discovery process.
iii) CRISPR-based hits validation
Target hits discovered through CRISPR-based screens are validated through a number of cell-based assays. CRISPR enables rapid, economical model generation through precise genome editing that accurately recapitulates the disease. CRISPR is widely used in disease model studies and helps in understanding the genetic basis of disease for development of better therapeutics. CRISPR technology also allows scientists to produce cell lines (immortal cell lines, primary cells and stem cells) and organoids with disease‑specific genetic backgrounds that have greatly improved the efficacy of hits validation. This robust and unbiased means of validating target hits improves success and accelerates development of therapeutics. It also helps in conducting basic research on disease-relevant cellular pathways.5 Thus, the ability to produce relevant disease models using CRISPR technology has enabled hit validation and accurate assessment of potential drugs for therapeutic benefits.
iv) CRISPR-based lead optimisation
CRISPR enables rapid, economical model generation through precise genome editing that accurately recapitulates the disease”
Target hit validation narrows down thousands of hits to several lead compounds that are optimised and tested for safety. Lead optimisation is the most important step in the drug-development process, which includes synthesis of lead molecules with improved potency, reduced off-target activities, increased chemical stability and less toxicity. CRISPR has accelerated the lead optimisation process through generation of cellular and animal models for specific human disease conditions, which can then be used to evaluate the efficacy and safety of drug candidates. CRISPR has revolutionised the generation of transgenic animals. For instance, mice models with multiple mutations in the genome can be easily generated using CRISPR in one step by editing genomes in zygotes, which circumvents the drawback of generating a model by crossing single mutant animals. CRISPR therefore offers the possibility of developing a realistic disease model for lead evaluation and optimisation.5
Precision therapeutics
The applications of CRISPR technology for precision medicine are exciting and wide ranging. Although this technology is still in its infancy, clinical development of CRISPR technology promises to change the way we think about monogenic and polygenic diseases. With the potential to repair faulty genes, CRISPR is one of the most anticipated technologies in modern therapeutics, promising to bring hope to disease sufferers.11,12 Recent advances in genetic and genomic technologies have paved the way for implementation of CRISPR in personalised medicine, where treatments are defined based on a patient’s genetic profile.13 Animal models have already demonstrated the successful use of CRISPR technology to correct hereditary tyrosinemia in a mouse liver. One approach to using CRISPR in precision therapeutics is to edit genomes of cells that can be removed from the body and then replaced, such as stem cells, immune cells and blood cells. However, there are many hurdles to be cleared before CRISPR can be successfully used in clinics for human therapeutics.14
Conclusion
CRISPR has gained increased attention following its introduction as a gene-editing tool in 2012 and is being widely used in the generation of cellular and animal disease models to accelerate drug discovery. Combined with other high-throughput technologies, CRISPR appears to open up the possibility of large-scale screening of drug targets towards advancing pharmacological research. CRISPR makes gene editing easier and more precise, therefore making drug target identification faster, which could speed up the drug discovery process. Biotech and pharmaceutical companies are also investing in CRISPR technology to build platforms for the development of next-generation therapeutics. In parallel, CRISPR also holds promise for developing faster therapies that could contribute toward advances in precision therapeutics. Thus, CRISPR technology serves as an important tool in drug discovery and this will ultimately pave the way for better and safer therapeutics.
About the author
Dr Pushpanathan Muthuirulan is currently a Research Associate at Harvard University studying the developmental and genetic basis of human height variations using functional genomics approaches. Previously, he worked as a Postdoctoral Researcher at the National Institutes of Health, where his research focused on developing state-ofthe- art technologies using CRISPR-Cas9 and super‑resolution microscopy to map neural circuits that involves visual motion information processing in Drosophila. His expertise lies in omics technologies, drug discovery, neuroscience and developmental and evolutionary genetics.
References
- Muthuirulan P. (2017). CRISPR-Cas9: Promising Platform for Prospective Antimicrobial Therapy. Adv Tech Clin Microbiol, 1(1).
- Mohs RC, Greig NH. (2017). Drug discovery and development: Role of basic biological research. Alzheimer’s & Dementia: Translational Research & Clinical Interventions, 3(4), 651-657.
- Van Norman GA. (2016). Drugs, devices, and the FDA: part 1: an overview of approval processes for drugs. JACC: Basic to Translational Science, 1(3), 170-179.
- Muthuirulan P. (2018). NGS: hunting mysterious ‘Dark Matter Genome’ towards rewriting the rules of human genetic diseases. Drug Target Review, 4(3), 72-75.
- Robert F, Huang S, Pelletier J. (2017). CRISPR/Cas9 Editing to Facilitate and Expand Drug Discovery. Current gene therapy, 17(4), 275-285.
- Jinek M, Chylinski K, Fonfara I, Hauer M, Doudna JA, Charpentier E. (2012). A programmable dual-RNA–guided DNA endonuclease in adaptive bacterial immunity. Science, 337(6096), 816-821.
- Baliou S, Adamaki M, Kyriakopoulos AM, Spandidos DA, Panayiotidis M, Christodoulou I, Zoumpourlis V. (2018). CRISPR therapeutic tools for complex genetic disorders and cancer. International journal of oncology, 53(2), 443-468.
- Lino CA, Harper JC, Carney JP, Timlin JA. (2018). Delivering CRISPR: a review of the challenges and approaches. Drug delivery, 25(1), 1234-1257.
- Lu Q, Livi GP, Modha S, Yusa K, Macarrón R, Dow DJ. (2017). Applications of CRISPR genome editing technology in drug target identification and validation. Expert opinion on drug discovery, 12(6), 541-552.
- Zhu S, Zhou Y, Wei W. (2017). Genome-wide CRISPR/Cas9 screening for high-throughput functional genomics in human cells. In Innate Antiviral Immunity (pp. 175-181). Humana Press, New York, NY.
- Hong A. (2018, December). CRISPR in personalized medicine: Industry perspectives in gene editing. In Seminars in perinatology(Vol. 42, No. 8, pp. 501-507). WB Saunders.
- Muthuirulan P, Gururajan V. (2017). CRISPR Inspires New Hope to Disease Sufferers. J Mole Microbiol, 2(1), 1.
- German DM, Mitalipov S, Mishra A, Kaul S. (2019). Therapeutic Genome Editing in Cardiovascular Diseases. JACC: Basic to Translational Science, 4(1), 122-131.
- Raikwar SP, Kikkeri NS, Sakuru R, Saeed D, Zahoor H, Premkumar K, Selvakumar GP. (2019). Next Generation Precision Medicine: CRISPR-mediated Genome Editing for the Treatment of Neurodegenerative Disorders. Journal of Neuroimmune Pharmacology, 1-34.
Related topics
CRISPR, Disease research, Drug Targets, Genome editing, Genomics, Research & Development, Screening
Related conditions
ancer, Cystic fibrosis, Haemophilia, Heart disease, hereditary tyrosinemia, HIV, Osteoarthritis, sickle cell disease