The elegant parallel of using CRISPR to understand disease mechanisms
Posted: 17 September 2019 | Dr Mark Behlke (Integrated DNA Technologies) | No comments yet
CRISPR is the buzzword of the moment in the drug discovery industry – mainly due to its potential to correct disease-causing mutations. However, those using the technology need to be mindful that it is used responsibly, and possible risks are considered before use. Mark Behlke discusses the potential of CRISPR in R&D and the challenges that it presents for researchers.
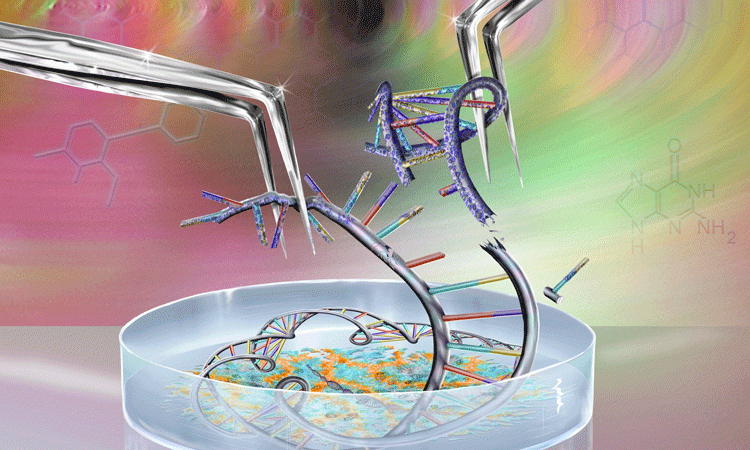
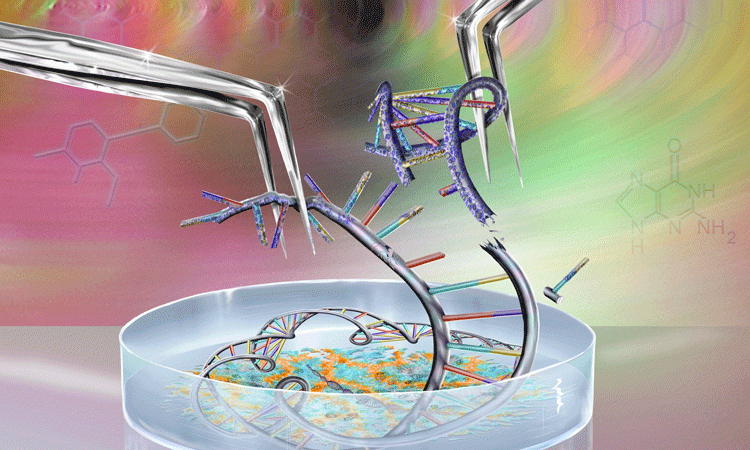
CRISPR TECHNOLOGY has generated much excitement in the drug discovery realm for its ability to make precise, permanent changes to DNA in animals, as first demonstrated approximately six years ago. It is currently being evaluated in early phase clinical trials for several disorders. Diseases caused by a single gene mutation – sickle cell disease (SCD), Huntington’s disease and cystic fibrosis – are all prime targets for using CRISPR gene therapy to correct the disease-causing DNA mutations. CRISPR is also being investigated as a treatment for acquired immune deficiency syndrome (AIDS) and to improve anti-tumour immunotherapy.
While news about potential CRISPR therapeutics and the start of new clinical trials dominate headlines in the lay press, CRISPR has also become a leading research tool to help scientists better understand gene function and establish model systems of human diseases needed to translate basic scientific knowledge into new medical treatments.
The potential of CRISPR
Use of recombinant Cas9 enzyme bound to a synthetic guide RNA as a ribonuclear protein (RNP) complex is one approach to performing gene editing that reduces OTE risks by limiting the duration of exposure of the CRISPR reagents to DNA. In fact, RNP methods will be used in numerous planned Phase I clinical trials in the coming years. While several Cas9 enzyme mutants have been described that improve specificity, they all show markedly reduced activity when used in the popular RNP format, thus limiting their utility for medical therapeutics. We have solved this problem by evolving a new mutant high-specificity Cas9 enzyme using a novel unbiased bacterial screening system. This new ‘HiFi’ Cas9 markedly reduces OTEs while retaining high activity comparable with wildtype Cas9 and is perfect for use in RNP applications. It is an optimised genome editing solution that outperforms other CRISPR-Cas9 systems for producing on-target, double-stranded DNA breaks. Given the highly active and specific nature of this high-fidelity Cas9 variant when delivered as an RNP complex, it is ideal for use in translational medical research and clinical trials.
Through this work, I have had the opportunity to collaborate with several researchers conducting cutting-edge investigations to better understand and ultimately cure a number of diseases. One such scientist is Dr Ayal Hendel, whose work has focused on developing gene-editing technology to provide cures for diseases such as Severe Combined Immunodeficiency (SCID), which is a group of rare life-threatening disorders caused by mutations in different genes involved in the development and function of immune cells. Dr Hendel believes that the ultimate cure for SCIDs will involve the autologous transplantation of stem cells, with the SCID-causing genetic error corrected ex vivo using CRISPR to create a healthy and normal immune system.
CRISPR gene editing is intended to make permanent modifications to DNA at a single, specific location”
His current strategy involves removing haematopoietic stem and progenitor cells (HSPCs), the bone marrow stem cells that generate red and white blood cells, from patients and editing them in the lab to correct the gene mutation. The cells would then be reintroduced to the patient and engrafted, with the expectation that by correcting the stem cells, normal daughter immune cells will be produced, ultimately curing the disease.
The beauty of this model is that, in theory, it allows patients to become their own donors; eliminating the need to find individual donors that are a suitable match for each individual patient. Additionally, the editing takes place ex vivo, which limits exposure of the CRISPR editing machinery to only the desired target cell population – ie, it is not a systemic therapy where the patient’s entire body is exposed to gene editing. The aim is that this process would be a one-off procedure with the benefit of providing a life-long cure. However, we are not there yet. Current research is focusing on pre-clinical experiments in disease models developed using HSPCs from patients and healthy controls. It is anticipated that these experiments will progress relatively quickly to clinical trials in patients. This promising research brings much needed hope to patients with SCID and their families.
Overcoming challenges associated with CRISPR
This model opens the door for personalised medicine therapies for a variety of genetic disorders. Since people can have different mutations that create the same disease outcome, this methodology would allow individuals to receive different synthetic nucleic acid constructs to treat their individual mutations – a possibility that still seems far-fetched but could revolutionise modern medicine. However, it is important to acknowledge the challenges that are associated with CRISPR, particularly regarding unintentional OTEs and the need for caution when utilising this technology.
CRISPR has the potential to alter genetic sequences and may provide novel therapies for patients, as well as address a broader range of needs over time. As this technology evolves, the design and implementation of safety measures is imperative. Novel techniques, tools and forethought regarding various applications of CRISPR are essential for the careful, considered and responsible use of this technology. In spite of recent advances, there remain concerns around OTEs. Care must be taken to predict and prevent inaccurate editing, especially when used or tested in human cells – both in vitro and in vivo. This should ensure that the power and promise of CRISPR technologies is realised in applications for good, both now and in the future.
About the author
Dr Mark Behlke is Chief Scientific Officer at Integrated DNA Technologies (IDT) and has directed many activities since joining the company in 1995, with a focus on novel molecular biology applications of oligonucleotide-based technologies. Before joining IDT, Dr Behlke was a Physician Postdoctoral Fellow of the Howard Hughes Medical Institute at the Whitehead Institute, MIT. He was a Resident Physician in Internal Medicine at Brigham and Women’s Hospital, Boston. Dr Behlke is an inventor on over 50 issued US patents, has numerous pending patent applications and is an author of over 130 scientific publications and book chapters.
Related topics
CRISPR, DNA, Genome editing, Genomics, Personalised Medicine, RNAs, Stem Cells
Related conditions
AIDS, Cystic fibrosis, Huntington's disease, Severe Combined Immunodeficiency (SCID), Sickle cell disease (SCD)
Related organisations
Bio-Rad
Related people
Dr Ayal Hendel