Heat shock proteins: A high content assay opportunity to measure cellular stress response
Posted: 10 May 2014 | Drug Target Review | No comments yet
Heat shock proteins (HSPs) have been proposed as therapeutic targets for novel anticancer agents. However, candidates have thus far failed due to low tolerability, limiting their efficacy. This failure highlights our current inability to predict drug toxicity in patients, a Holy Grail in modern drug discovery. Paradoxically, the fundamental role of the tightly regulated HSPs in maintaining homeostasis may constitute an opportunity to monitor drug toxicity. We propose to incorporate the quantification of HSP induction as a key indicator of cellular stress response in multiplexed, high content cell based assay readouts to enhance the predictive power of in vitro drug toxicity profiling outcome.
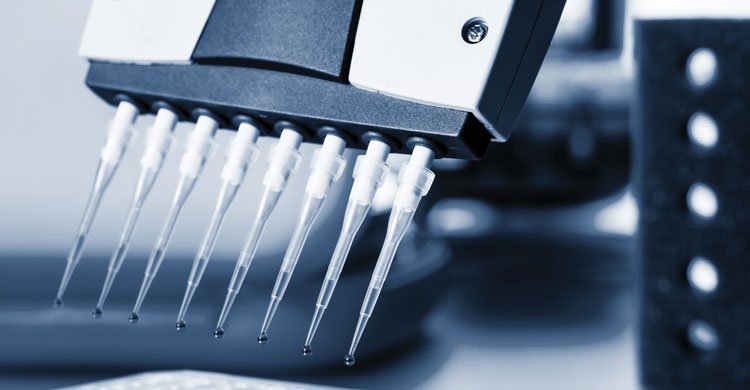
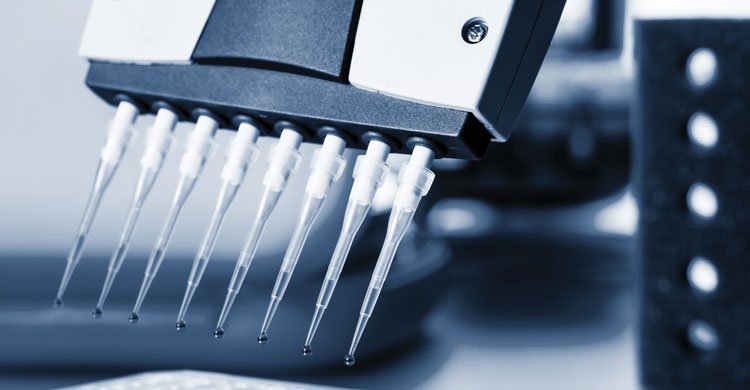
HSPs as essential cell guardians of proteome integrity
With the complexity of proteome networks and a crowded cellular environment with an estimated total protein concentration of 300 mg/mL, maintaining correct protein folding under these conditions constitutes a challenge for the cell1. In addition, four main conditions routinely trigger protein aggregation under normal physiology, thereby threatening homeostasis; mutations, defects in protein biosynthesis, aging and environmental stress2. The accumulation of misfolded proteins constitutes a serious threat to the cell by leading to protein aggregation perturbing normal cell function, ageing and various other disorders2. Consequently, cells have evolved early on to help correct folding of nascent proteins and to prevent the accumulation of misfolded proteins by relying on molecular chaperones1,2.


Table 1: Summary table of the main families of heat shock proteins, their described cellular location and proposed function
Among the main molecular chaperones are HSPs upregulated in response to stress such as heat and play important physiological roles in normal and under systemic and cellular stress conditions3. HSPs belong to five main families according to their respective molecular weight and have distinct cellular localisations and proposed functions. Owing to their fundamental role in maintaining homeostasis, HSPs have been found to be expressed in most cell compartments to accomplish various functions (Table 1)1-9. Interestingly, cell stress may trigger HSP translocation following induction of expression, such as HSP10 which is mainly localised in the mitochondrial matrix under normal conditions but migrates to the cytosol in response to stress4. In addition, we have observed HSP10 translocation to the nucleus under toxic conditions (Figure 1A and 1B), consistent with previous observations for other small HSPs3.


Figure 1: Microscopy images illustrating the cellular location of HSP10 (A&B), HSP60 (C), and HSP70 (D) in HeLa control untreated cells (A) or transfected with an EGFP plasmid using Dharmafect1 chemical transfection reagent (B-D). Cells were imaged using the INCell Analyzer 6000 automated epifluorescence microscope with confocal modality (INCA6000; GE Healthcare) at 40X objective magnification and the fused images were acquired in the blue channel for Hoechst staining of nuclei, green channel for EGFP expression, red channel for HSP immunostaining and far red channel for microtubule staining with phalloidin (depicted in grey).
The inducible expression of HSPs is regulated by constitutively expressed Heat Shock Factors (HSFs)10. The mechanisms and molecular events responsible for the activation of HSP expression are complex, unclear and are believed to involve interplay, crosstalk and post-translational regulation10. Not surprisingly, a highly conserved and tightly regulated system in the cell is dedicated to preserving proteome integrity; in fact, heat shock response represents one of the most ancient molecular pathways and is conserved among many species9. These observations highlight the fundamental role of HSPs in maintaining cell homeostasis.
HSPs as targets for anticancer therapeutic agents
Increased expression of HSPs has been commonly observed in human cancers and HSP overexpression generally correlates with poor prognosis11. For this reason, HSPs and especially HSP90 have been considered as potential targets for cancer therapy. HSP90 is being actively pursued as a target for novel anticancer agents12-14 based on the observation that the benzoquinone geldanamycin can revert v-SRC mediated cell transformation by inhibiting the formation of the active heterodimer formed with this HSP90 client15. However, HSPs including HSP90 are ubiquitous and constitute up to two per cent of the total cellular protein content1,11, and this amount increases by a further two-fold upon induction11. In addition, HSP90 over-expression likely reflects a protective stress response to the hypoxic, acidic and nutrient-deprived tumour microenvironment11. Nevertheless, HSPs are being considered as drug targets for novel anticancer agents based on the hypothesis that malignant cells have heightened dependence on the maintenance of intracellular homeostasis of rapidly dividing cells in a nutrient-deprived environment, and dependence on key client proteins11-14. Indeed, many oncogenes have been identified among HSP90 clients, such as ERBB2/HER2, ALK and BRAF13, but many other proteins are also among the ~200 identified clients of the highly conserved HSP90 machinery. Targeting HSP90 that regulates extraordinarily diverse cellular processes (including innate and adaptive immunity, protein trafficking and RNA processing)14 is at risk of yielding undesirable effects upon inhibiting its function; since not surprisingly, HSP90 expression has been found to be necessary to the survival of all eukaryotes tested to date14.
Thus far, approximately 20 HSP90 inhibitors (HSP90is) have entered clinical trials, and despite little or no clinical response, issues limiting their use are emerging. To date, no HSP90i has been approved by the FDA, despite many years of trials and tribulations16. The development of tanespimycin was halted by Bristol-Myers Squibb (BMS) after Phase I and II studies14-17. Retaspimycin showed an overall objective response rate of seven per cent in patients with molecularly defined non-small cell lung cancer (NSCLC), well below the threshold success rate of 20 per cent14,18. Moreover, a recent multi-centre study involving retaspimycin in combination with trastuzumab in patients with advanced or metastatic HER2-positive breast cancer patients showed no confirmed responses at the dosages tested19. Dose limiting toxicity including diarrhoea, fatigue and ocular effects was observed with the resorcyclicisoxazole NVP-AUY922 in Phase I/II clinical trials in patients with NSCLC, breast and gastric cancers14. Dose limiting toxicity including syncope and dizziness was also observed in patients with advanced solid tumours treated with the purine scaffold drug BIIB021 currently developed by Premiere Oncology14,20. A Phase II study of BIIB021 in refractory GIST patients showed a partial response by FDG-PET21. Pfizer also halted the development of the 2-aminobenzamide compound PF04929113 (previously SNX-5422) due to ocular toxicity observed in a Phase I trial14,22.
The failure in the clinic of HSP90is illustrates the challenge of targeting and developing HSP inhibitors as anticancer drugs. Data from preclinical studies in mouse models show that a cytostatic effect as opposed to tumour regression; which is typically observed as a favourable outcome23, which is not what is expected from the supposed mechanism of action14. In general, a lack of efficacy due to low tolerability limits current HSP90is development. To date, encouraging clinical data has only been observed in specific cancers, such as HER2-positive breast cancer and ALK-rearranged NSCLC14. Overall, the difficulty to predict responders11 and the resulting difficult patient selection remain the unattainable challenges24; not consistent with the clinical response expected from the supposed dual mechanism of action of HSPi targeting simultaneously multiple oncogenes and inducing proteotoxic stress. For example, it is unknown why a clear benefit in BRAF melanoma patients has not been observed so far, given the dependence of client proteins on HSP9016. This failure in the clinic raises the question: does targeting HSPs that are highly conserved and essential to all eukaryotes constitute a flawed concept? HSPs are not different in cancer cells and their expression level is similar in both normal and cancer cells, which probably explains the limited tolerability of HSPis. As pointed out by others, the role of HSP90 in normal physiology may prove to be problematic16.
Among the next steps considered is the exploration of drug combinations, but with multiple HSPis or with agents with an alternative mechanism of action (MOA). Combining multiple HSPis raises the risk to reduce tolerability even further, the toxicity of such combinations being unclear16. Combining HSPis with inhibitors of client proteins, on the other hand, may well succeed under some conditions. Another approach consists in the development of second generation inhibitors; however, the advantages and benefits of developing more specific inhibitors remain unclear, when HSPis will affect multiple HSP clients to begin with.
HSPs as indicators of cellular stress response in drug discovery
The failure in the clinic of drug candidates targeting HSPs illustrates the current greatest challenge in drug discovery: to translate in vitro observations into a successful drug in patients. Most often, the toxicity of small molecules limits their efficacy but the lack of predictive tools for in vivo toxicity makes it difficult to successfully prioritise candidates from hits emerging from high throughput screening efforts. Current cytotoxicity assays have limitations, most notably the inability to monitor simultaneously all the various and complex aspects of cell death accurately and the propensity to overlook early signs of toxicity25,26; as a result few predictive systems are of value so far27. The ultimate goal in drug discovery is the ability to predict the in vivo toxicology of drug candidates based on in vitro cytotoxicity profiling26.
Paradoxically, the failure of drugs targeting HSPs may provide an opportunity to enhance the predictive power of in vitro drug toxicity profiling outcome by highlighting the fundamental and highly conserved role of this stress response. We have recently demonstrated that HSP induction could be used to monitor cell stress induced upon chemical transfection28. In an attempt to identify optimal transfection conditions, we measured by immunostaining and automated microscopy image analysis the induction of HSP expression in response to commercial chemical formulations used to transfect nucleic acids. For example, upon transfection of an EGFP plasmid using Dharmafect1, we observed induction of HSP10 expression in the cytosol and nucleus compared to control (Figure 1A and 1B), a strong induction of HSP60 expression in the cytosol and mitochondria (Figure 1C) and induction of HSP70 expression in the cytosol and nucleus (Figure 1D). These observations, are consistent with previously described cellular locations for these HSPs (Table 1), and highlight the importance of monitoring HSP induction as a measure of cell stress/fitness to capture the full spectrum of cytotoxic effects induced by small molecules.
Following up on these observations, we hypothesised that HSP induction could be used to monitor cell stress induced by drug candidates. To test this hypothesis, we profiled a panel of metalloprotease inhibitors for HSP60 induction as a time course for three different compound concentrations (Figure 2A). We compared HSP induction with cell proliferation by extracting nuclei count from the same wells (Figure 2B). Included in the panel were inhibitors of human peptide deformylase (HsPDF), a metalloprotease thought to be the target responsible for the antiproliferative effect of actinonin (Figure 2C)29-30. In addition to the well-characterised broad spectrum metalloprotease inhibitor actinonin31, we included three compounds belonging to the benzofurane-diones chemical class, a new class of non hydroxamic acid based, non-eptidomimetic based PDF inhibitors that we have shown to be selective for HsPDF32: BF-13, SKI 417516 and SKI 417520 (Figure 2C). Background HSP60 induction was observed for DMSO controls, mostly for the 24 hour time point, likely reflecting cellular stress during the mechanical cell seeding step. As expected, this induction decreased over time for DMSO controls while the nuclei count increased as cells proliferated after seeding. The HSP60 induction profile we captured mirrored the antiproliferative effect of all compounds of the panel, with high HSP60 induction being associated with low nuclei count (Figure 2A and 2B). For example, at 48 hours post treatment, low HSP60 induction was observed for DMSO control wells, correlating with high nuclei count; in contrast, high HSP60 induction was observed for cells treated with actinonin, correlating with low nuclei count (Figure 2A- 2C). Of note, HSP60 expression induced by actinonin co-localised in part with Mitotracker staining of mitochondria and was also observed in the cytosol (Figure 2C), as expected (Table 1). Significant HSP60 induction compared to controls was observed for the cell-potent HsPDF inhibitors actinonin, BF-13 and SKI 417516, while no induction was observed for the HsPDF inhibitor SKI 417520 (Figure 2A). This observation tends to demonstrate the specificity of our HSP induction readout.


Figure 2A: Bar graph quantification of HSP60 induction in HeLa cells by a panel of metalloprotease inhibitors tested at 1, 10 and 50 µM after 24, 48 and 72 hour incubation, including HsPDF inhibitors actinonin, BF-13, SKI 417516 and SKI 417520.
Furthermore, significant HSP60 induction by the HsPDF inhibitors was observed at 24 hours post-treatment, while only a small difference in cell count could be detected at this time point. This result indicates that HSP induction could be useful in predicting small molecule toxicity. Interestingly, BF-13 at the highest concentration was the only compound to strongly induce HSP60 expression at the 24-hour time point. This observation correlates with the fact that BF-13 was cytotoxic as early as 24 hours, as opposed to being cytostatic like the other HsPDF inhibitors (Figure 2B). In addition, we found that among our panel of metalloprotease inhibitors, only the cell-potent PDF inhibitors and not the other metalloprotease inhibitors induced sustained HSP60 expression over 72 hours. Strikingly, treatment with the three HsPDF inhibitors yielded very similar phenotypes, both in terms of HSP60 induction and morphology. This was true even when comparing actinonin with BF-13 and SKI 417516, despite the fact that actinonin belongs to a completely different chemical class (Figure 2C). Therefore, our results strongly suggest that small molecules sharing a common mechanism of action maybe characterised by a similar HSP induction profile. Altogether, our observations indicate that monitoring HSP induction might prove useful in characterising and predicting the cell-based mechanism of action of small molecules, and highlight the power of high content imaging for that purpose.


Figure 2B: Bar graph quantification of nuclei count upon treatment with the panel of metalloprotease inhibitors in the same wells.
Monitoring HSP induction as both a qualitative and quantitative readout offers many advantages in the context of preclinical drug discovery. A qualitative readout such as which HSP is induced or its intracellular location upon induction may provide clues about the mechanism of action leading to toxicity (Table 1, Figure 1), while a quantitative aspect such as the level of induction allows to measure a spectrum of responses as opposed to binary readouts such as live or dead (Figure 2A). Combined with multiple time points for readout, monitoring HSP induction as a measure of cell stress may allow to detect early morphological or phenotypic changes that lead to cytotoxicity, as exemplified by HSP60 induction triggered 24 hour post treatment with the HsPDF inhibitor BF-13 (Figure 2A).


Figure 2C: Representative epifluorescence microscopy images captured using the INCA6000 at 40X magnification after 72 hour incubation with DMSO control (A), or with 50 µM of the HsPDF inhibitors actinonin (B), BF-13 (C) or SKI 417516 (D). The fused images were acquired in the blue channel for Hoechst staining of nuclei, green channel for Mitotracker staining of mitochondria, red channel for HSP immunostaining and far red channel for microtubule staining with phalloidin (depicted in grey).
In addition, more and more evidence shows that reactive drug metabolites covalently bind to cellular proteins and significantly contributes to drug toxicity. However, covalent binding assays are not typically conducted early on due to the need for radiolabeled drugs27. Monitoring HSP induction may constitute a good surrogate to detect protein adduct formation responsible for the in vivo toxicity of drugs. This example highlights how measuring stress induction may allow predicting adverse effects more accurately.
Additionally, assessing HSP induction may allow screening for chemical compounds that induce immunogenic cell death, highly desirable as anticancer agents because of their ability to recruit the immune system to contribute to tumour regression8,33. This is due to the fact that HSPs can stimulate the immune system by cross-presenting peptide-HSP complexes, sometimes in response to small molecules such as upon treatment with the proteasome inhibitor bortezomib, triggering HSP90 to be presented at the cell surface33.


Figure 3: Proposed multiplexed readout for a more accurate assessment of drug toxicity.
From the same well, a measurement of metabolic activity, cell proliferation, induction of apoptosis and cell stress provides an exhaustive and accurate report on cellular health, likely to enhance the predictive power of in vitro cytotoxicity profiling.
Conclusion
As anticancer drug targets, HSPs have yet to yield a drug successful in the clinic. As early reporters of cell stress, HSPs could constitute powerful tools to enhance the predictive profiling power of in vitro drug toxicity screening. For this purpose, we propose to monitor HSP induction as the fourth leg of a multiplexed assessment of drug toxicity. From the same well, a measurement of metabolic activity, cell proliferation, induction of apoptosis and cell stress provides an exhaustive spectrum and accurate report on cellular health (Figure 3). HSPs as indicators of cellular stress response may help reaching the ultimate goal of preclinical drug discovery; to better prioritise drug candidates and potentially reduce attrition in the clinic.
Acknowledgement
The authors wish to thank the members of the HTS Core Facility for their help during the course of this study. The HTS Core Facility is partially supported by Mr. William H. Goodwin and Mrs. Alice Goodwin and the Commonwealth Foundation for Cancer Research, the Experimental Therapeutics Center of the Memorial Sloan-Kettering Cancer Center, the William Randolph Hearst Fund in Experimental Therapeutics, the Lillian S. Wells Foundation, and by a NIH/NCI Cancer Center Support Grant 5 P30 CA008748-44.
References
- Taipale, M. et al. (2010) HSP90 at the hub of protein homeostasis: emerging mechanistic insights. Nat Rev Mol Cell Biol 11 (7), 515-528
- Tyedmers, J. et al. (2010) Cellular strategies for controlling protein aggregation. Nat Rev Mol Cell Biol 11 (11), 777-788
- Kregel, K.C. (2002) Heat shock proteins: modifying factors in physiological stress responses and acquired thermotolerance. J Appl Physiol 92 (5), 2177-2186
- David, S. et al. (2013) Hsp10: anatomic distribution, functions, and involvement in human disease. Front Biosci (Elite Ed) 5, 768-778
- Koll, H. et al. (1992) Antifolding activity of hsp60 couples protein import into the mitochondrial matrix with export to the intermembrane space. Cell 68 (6), 1163-1175
- Kourtis, N. and Tavernarakis, N. (2011) Cellular stress response pathways and ageing: intricate molecular relationships. EMBO J 30 (13), 2520-2531
- Martin, J. et al. (1992) Prevention of protein denaturation under heat stress by the chaperonin Hsp60. Science 258 (5084), 995-998
- Srivastava, P. (2002) Roles of heat-shock proteins in innate and adaptive immunity. Nat Rev Immunol 2 (3), 185-194
- Velichko, A.K. et al. (2013) Mechanisms of heat shock response in mammals. Cell Mol Life Sci
- Akerfelt, M. et al. (2010) Heat shock factors: integrators of cell stress, development and lifespan. Nat Rev Mol Cell Biol 11 (8), 545-555
- Whitesell, L. and Lindquist, S.L. (2005) HSP90 and the chaperoning of cancer. Nat Rev Cancer 5 (10), 761-772
- Jhaveri, K. and Modi, S. (2012) HSP90 inhibitors for cancer therapy and overcoming drug resistance. Adv Pharmacol 65, 471-517
- Patel, H.J. et al. (2011) Advances in the discovery and development of heat-shock protein 90 inhibitors for cancer treatment. Expert Opin Drug Discov 6 (5), 559-587
- Travers, J. et al. (2012) HSP90 inhibition: two-pronged exploitation of cancer dependencies. Drug Discov Today 17 (5-6), 242-252
- Whitesell, L. et al. (1994) Inhibition of heat shock protein HSP90-pp60v-src heteroprotein complex formation by benzoquinone ansamycins: essential role for stress proteins in oncogenic transformation. Proc Natl Acad Sci U S A 91 (18), 8324-8328
- Hong, D.S. et al. (2013) Targeting the molecular chaperone heat shock protein 90 (HSP90): lessons learned and future directions. Cancer Treat Rev 39 (4), 375-387
- Arteaga, C.L. (2011) Why is this effective HSP90 inhibitor not being developed in HER2+ breast cancer? Clin Cancer Res 17 (15), 4919-4921
- Sequist, L.V. et al. (2010) Activity of IPI-504, a novel heat-shock protein 90 inhibitor, in patients with molecularly defined non-small-cell lung cancer. J Clin Oncol 28 (33), 4953-4960
- Modi, S. et al. A multicenter trial evaluating retaspimycin HCL (IPI-504) plus trastuzumab in patients with advanced or metastatic HER2-positive breast cancer. Breast Cancer Res Treat 139 (1), 107-113
- Jhaveri, K. et al. (2012) Advances in the clinical development of heat shock protein 90 (Hsp90) inhibitors in cancers. Biochim Biophys Acta 1823 (3), 742-755
- Dickson, M.A. et al. Phase II study of the HSP90-inhibitor BIIB021 in gastrointestinal stromal tumors. Ann Oncol 24 (1), 252-257
- Rajan, A. et al. (2011) A phase I study of PF-04929113 (SNX-5422), an orally bioavailable heat shock protein 90 inhibitor, in patients with refractory solid tumor malignancies and lymphomas. Clin Cancer Res 17 (21), 6831-6839
- Workman, P. et al. (2007) Drugging the cancer chaperone HSP90: combinatorial therapeutic exploitation of oncogene addiction and tumor stress. Ann N Y Acad Sci 1113, 202-216
- Scaltriti, M. et al. (2012) Molecular pathways: targeting hsp90–who benefits and who does not. Clin Cancer Res 18 (17), 4508-4513
- Kepp, O. et al. (2011) Cell death assays for drug discovery. Nat Rev Drug Discov 10 (3), 221-237
- Ramirez, C.N. et al. (2010) Cell viability assessment: toward content-rich platforms. Expert Opin Drug Discov 5 (3), 223-233
- Liebler, D.C. and Guengerich, F.P. (2005) Elucidating mechanisms of drug-induced toxicity. Nat Rev Drug Discov 4 (5), 410-420
- Antczak, C. et al. (2013) A High Content Assay to Assess Cellular Fitness. Comb Chem High Throughput Screen (In Press)
- Antczak, C. et al. (2007) High-throughput identification of inhibitors of human mitochondrial peptide deformylase. J Biomol Screen 12 (4), 521-535
- Lee, M.D. et al. (2003) A new human peptide deformylase inhibitable by actinonin. Biochem Biophys Res Commun 312 (2), 309-315
- Antczak, C. et al. (2008) A profiling platform for the identification of selective metalloprotease inhibitors. J Biomol Screen 13 (4), 285-294
- Antczak, C. et al. (2011) Identification of benzofuran-4,5-diones as novel and selective non-hydroxamic acid, non-peptidomimetic based inhibitors of human peptide deformylase. Bioorg Med Chem Lett 21 (15), 4528-4532
- Zitvogel, L. et al. (2008) Immunological aspects of cancer chemotherapy. Nat Rev Immunol 8 (1), 59-73
About the authors
djaballh@mskcc.org