The role of biomaterials in stem cell-based regenerative medicine
Posted: 6 June 2018 | Laura Goldie - Head of Technical Services - Biogelx Ltd. | 3 comments
Despite their vast therapeutic potential in such areas as cell therapy and tissue engineering, stem cells have yet to live up to their original hype and demonstrate widespread clinical success.
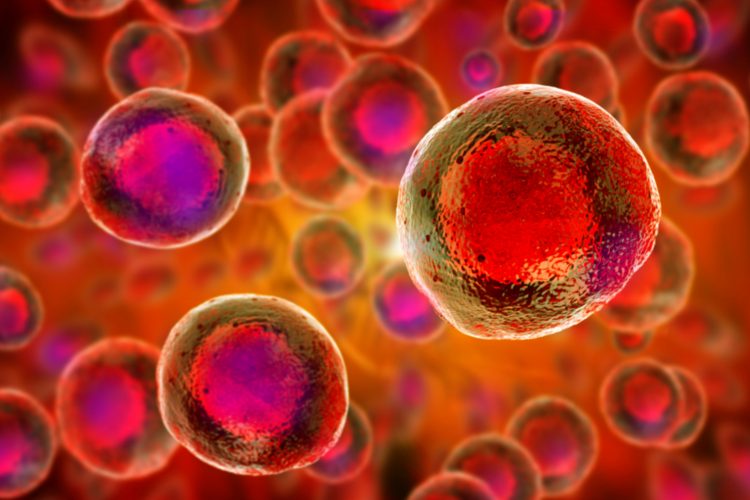
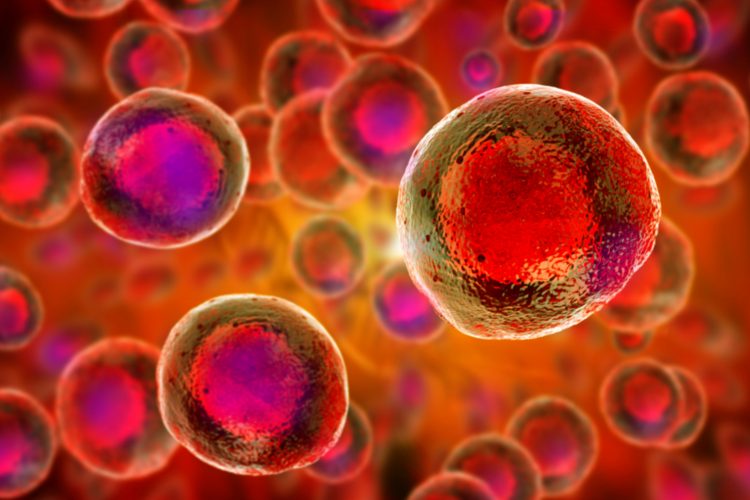
There is only one FDA-approved stem cell treatment, that being the use of blood-forming stem cells derived from cord blood, which have been used for the treatment of leukaemia for decades. Despite the recent rapid advances within the field of stem cell research, and an ever-growing population of researchers in academia, biotech, and the pharmaceutical industry focused on the development of stem cell-based treatments for a range of conditions, there has been a limited progression of such research from bench to bedside.
The lack of success in translation of stem cell technologies to clinical applications can be attributed to a range of issues, from the well-documented ethical, legal, and social controversies which surround the use of certain stem cells, to practical obstacles such as challenges in obtaining a sufficient quantity of cells required for clinical applications, and issues in achieving efficient and uniform differentiation of stem cells into functional derivatives for the regeneration of damaged tissues. As our understanding of stem cells and their interaction with their environment develops, it has become apparent that the use of biomaterials to improve stem cell cultures and control stem cell behaviour will be vital for cellular therapy and tissue regeneration.
The combination of an aging population, demand for a higher quality of life, and the lack of any effective therapies or cures for the treatment of spinal cord or traumatic brain injuries, or neurodegenerative diseases like Alzheimer’s disease and Parkinson’s disease, has led to the emergence of regenerative medicine and tissue engineering as a solution. With their ability to self-renew and differentiate into specialised cell types, the interest in utilising stem cells for the regeneration of damaged / lost tissue and organs has intensified. There are three types of stem cells; embryonic stem cells (ESCs) and induced pluripotent stem cells (iPSCs) are capable of unlimited self-renewal and can differentiate into any of the over 200 types of cells in the body. The third, adult stem cells, which include mesenchymal stem cells (MSCs) are isolated from various sources, such as bone marrow and adipose tissues, and are multipotent ie, they differentiate into only limited cell types. Whilst ESCs and iPSCs can pose issues due to uncontrolled differentiation and tumorigenicity, and the ethical concerns surrounding their use, adult stem cells like MSCs are not plagued with such concerns. Based on this and their relative ease of culture, MSCs are one of the most widely studied stem cells in regenerative medicine.
MSCs exist in almost all types of tissues. They possess a multilineage potential, with their capacity to self-renew and differentiate into specialised cell types being dependant on their source of isolation. Previously it has been demonstrated that they are able to differentiate into adipocytes, chondrocytes and osteocytes, myoblasts, cardiomyocytes and neurons in vitro and in vivo. In addition to their differentiation capabilities, lack of ethical concerns, and no reported tumorigenicity, the wide availability of autologous sources and emerging evidence that they are immunosuppressive make MSCs even more attractive candidates for regenerative medicine applications.
For the successful incorporation of MSCs into cell therapy and tissue engineering applications, a thorough understanding of the factors which influence their behaviour is vital. Their fate decisions are driven by various instructive factors from their immediate vicinity or microenvironment. These biochemical and biophysical cues play a key role in determining the efficacy of MSC differentiation and thus their contribution to the repair process. The ability to tailor such cues and incorporate them into the design of specific 3-D microenvironments would allow the direction of stem cell fate and potentially optimise and facilitate tissue repair / regeneration. It is in this context, that an understanding of materials science and design becomes important, in allowing the creation of biomaterials that possess the optimal biochemical and mechanical properties required to manipulate the stem cells for specific therapeutic applications. Indeed, it is believed by many that due to low proliferation rates and limited lifespan, MSCs alone would not be able to meet the demands of tissue engineering / regenerative therapies and that strategies which exploit the interaction of MSCs and biomaterials will be vital in translating this stem cell research to the clinic.
It is known that the most significant element in regulating MSC fate is the ECM. Stem cells are attached to the ECM via adhesion molecules, and cell-ECM interactions play a key role not only in cell adhesion, but cell morphology, cell-cell interactions, and differentiation. This interaction between stem cells and biomaterials is the key basis for influencing stem cell properties in vitro or in vivo. As such, within tissue engineering, biomaterials should be designed to mimic the biological and physical properties of the microenvironment of native ECM found in tissues, thereby attempting to recreate the native niche of stem cells or MSCs derived from particular sources.
Biomaterial scaffolds should provide mechanical and 3D structural support for cells, present the appropriate biochemical features to support cellular function, and of course in the context of clinical applications not induce inflammatory responses.
In terms of classes of biomaterial, both natural and synthetic biocompatible and biodegradable materials can provide various biological signals and differing degrees of mechanical strength. With the utilisation of natural biomaterials (eg, collagen, alginate, chitosan) there is an inherent bioactivity, however, the ability to tune the properties of such materials is limited. In addition to this, animal-derived materials often have issues of lot-to-lot variability and the potential of xenogenic components to transmit pathogens / disease which would limit their use in the clinic. Synthetic polymers (eg, polyethelene glycol (PEG), poly-lactic acid (PLA)) on the other hand can be manufactured with high reproducibility, and characteristics such as the mechanical properties and degradation profile can be more easily controlled. A disadvantage to many synthetic materials is the lack of cell adhesion and recognition sites. Furthermore, with the exception of materials such as PEG which has been shown to be safe for biomedical applications in humans, the biocompatibility of synthetic ECM mimics is called into question upon transplantation in vivo.
When designing a biomaterial to manipulate MSCs (or other stem cells) for regenerative medicine applications, the structure and morphology of the material, in addition to biological properties such as wettability, chemical functionality, material biodegradability, and biophysical properties such as matrix stiffness and strength are all important factors that may significantly influence cell behaviour. Whether utilising a naturally-derived biomaterial, designing a novel synthetic material, or developing a combination material incorporating both natural biomaterial and synthetic polymer, the following properties will play a key role in dictating stem cell fate:
- Wettability – It is known that proteins will adsorb in different ways onto biomaterial surfaces depending of the degree of hydrophobicity / hydrophilicity (wettability) of the materials, which will in turn have an influence on cell adhesion and proliferation. As such an understanding of this property is crucial in order to modulate the biological response of stem cells.
- Chemical functionality – The ability to modify the chemistry of the biomaterial provides the opportunity to influence the surface characteristics and therefore the biomaterial-stem cell interactions. In addition to having an effect on cell adhesion, optimising the chemical functionality of a biomaterial also allows the incorporation of chemical cues in order to influence stem cell differentiation and offers the ability to incorporate specific biofunctionality in order to elicit a specific response from the cells.
- Matrix stiffness – Stem cells are known to be mechanosensitive. Extensive studies, most of these using MSCs, have demonstrated the response of stem cells towards the mechanical properties (stiffness) of a biomaterial. Matrix stiffness has been shown to regulate cell morphology, cellular functions, and differentiation capacity.
- Degradation – In the context of regenerative medicine applications, it is generally accepted that biomaterials serve as a temporary scaffold and, as new tissue is formed, they should degrade at a suitable and controllable rate with by-products that are non-toxic. It is necessary to design materials that degrade over a timescale that corresponds with a given stem cell application and allow for cell stretching, and ECM remodelling. Ideally, the degradation profile of the biomaterial will mirror concurrent synthesis of ECM by the cells.
Defined biomaterial scaffolds, in which the above chemical and physical properties have been optimised are of vital importance for current and future studies in controlling stem cell behaviour to maximise their full potential in regenerative medicine and tissue engineering. In line with this synergistic approach of combining biomaterials and stem cells, Biogelx is working with stem cell researchers on a broad range of applications. The company’s peptide hydrogel technology can easily be chemically and mechanically modified to mimic different tissues and meet the requirements of a range of cell types and have already been shown to promote cell adhesion, growth and proliferation in a range of applications including guiding the targeted differentiation of MSCs. It is envisaged that employing such simple, modifiable and reproducible materials, capable of being produced on the scale, will accelerate the translation of stem cell research from the bench to the clinic.
Biography
DR LAURA GOLDIE, Head of Technical Services, Biogelx Ltd. is a technical champion and an expert in Biogelx’s range of simple, yet functional peptide hydrogels and their varied applications. In this role, she uses her research background and commercial experience to support the development of products for specific cell-based applications by managing relationships with academic and industry partners.
The rest of this content is restricted - login or subscribe free to access
Why subscribe? Join our growing community of thousands of industry professionals and gain access to:
- quarterly issues in print and/or digital format
- case studies, whitepapers, webinars and industry-leading content
- breaking news and features
- our extensive online archive of thousands of articles and years of past issues
- ...And it's all free!
Click here to Subscribe today Login here
Related topics
Regenerative Medicine, Research & Development, Stem Cells
Related organisations
Biogelx Ltd
Interesting information, thank you for share, it helps me with my essay
True, FDA has only approved treatments of stem cells derived from cord blood and harvesting from fat is illegal.
It is amazing that we are not yet able to sort out the reactivity of allo or even auto antigens to make stem cell therapy a clinical success, I see there are groups of people sponsoring Animal stem cells for therapy in several countries where there are minimal or no regulatory controls. Wonder if there should be an international control to stop such bad actors whow are exploiting desperate patients.